Principles
Master the principles of High Performance Liquid Chromatography (HPLC) with our in-depth eLearning courses. Explore key chromatographic parameters like retention time, resolution, and efficiency. Understand column chemistry, including key properties of columns such as, particle size, length, diameter, and surface modifications. Learn how band broadening affects peak shape and separation quality, and discover strategies to minimize it. Optimize your mobile phase by balancing solvent composition, pH, and gradient elution for precise, reproducible results. Whether you're in pharmaceuticals, biotechnology, food safety, or environmental analysis, our expert-led training helps you enhance your analytical skills. Get hands-on with interactive content, real-world examples, and quizzes. Start learning today and elevate your HPLC expertise!
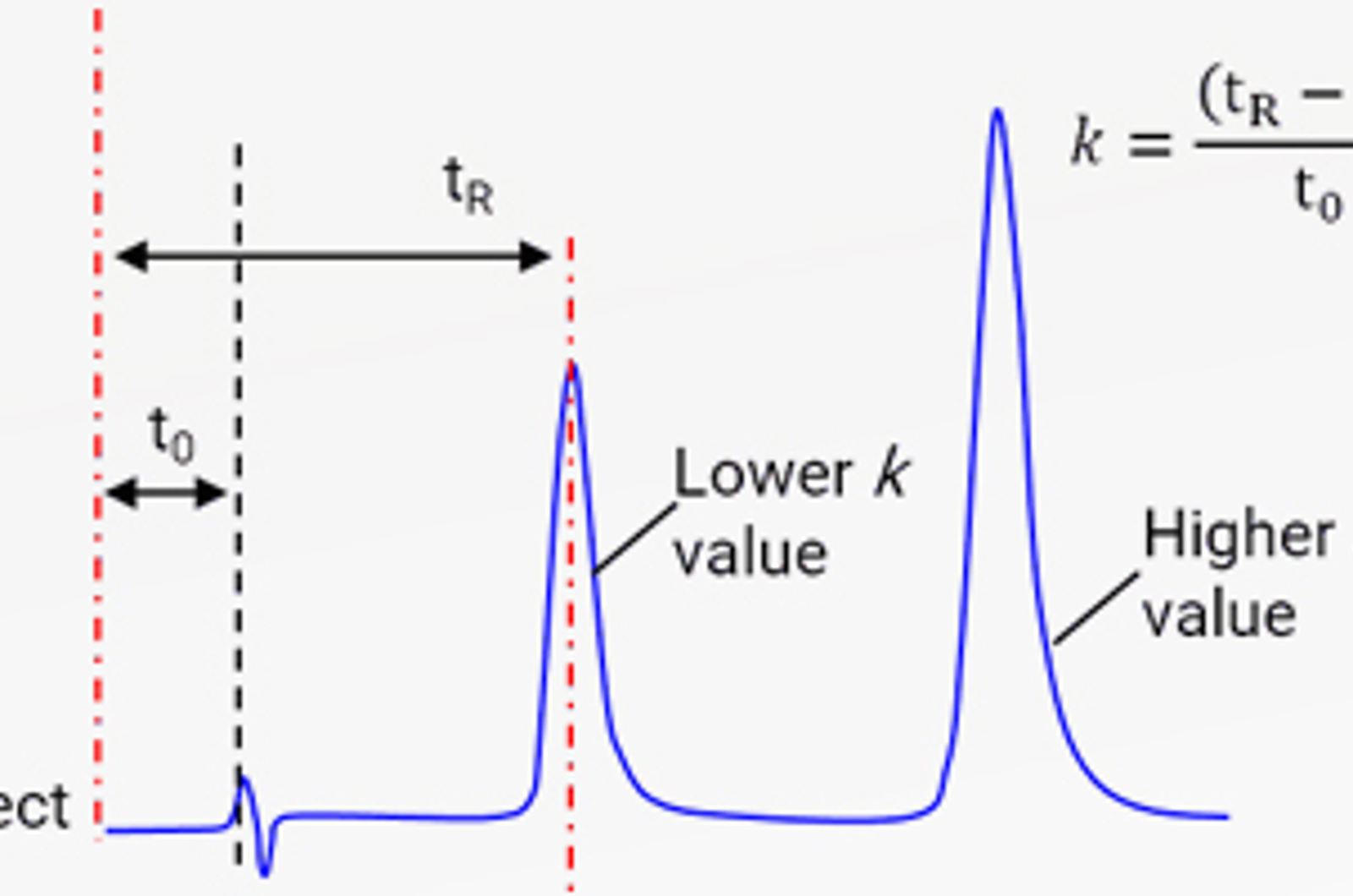
HPLC Chromatographic Parameters
The aim of this module is to introduce and explain the concept of chromatographic resolution. The resolution equation will be defined and its dependence on the chromatographic parameters retention factor, selectivity, and efficiency illustrated.
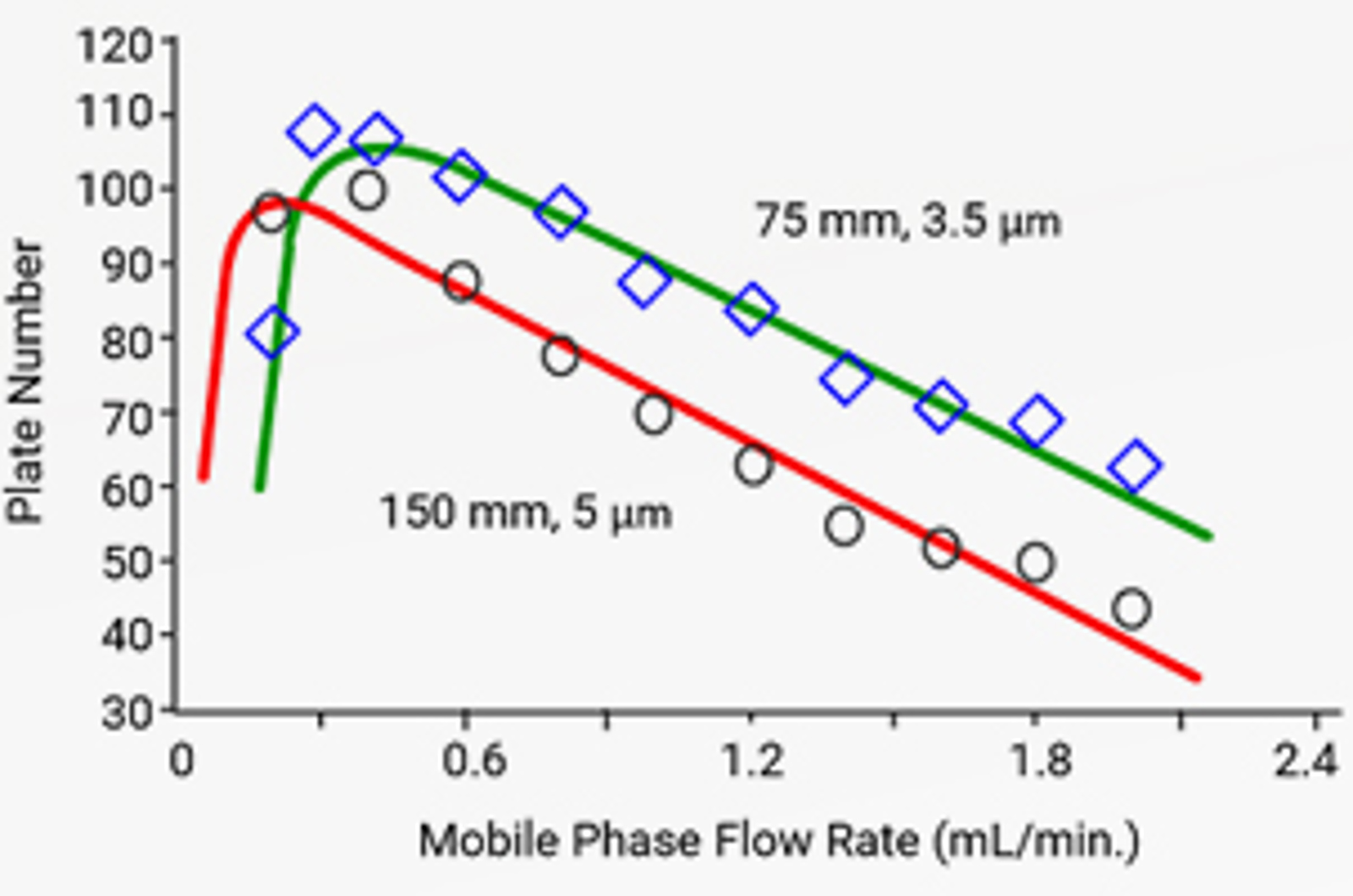
HPLC Column Chemistry
The aim of this module is to introduce silica as a major stationary phase support for reversed phase HPLC separations and for normal phase applications. The use of bonded phases in various chromatographic applications, and the influence of surface silica silanol groups on chromatographic separations is illustrated. Modern bonded phases that give an advantage to today’s chromatographer are introduced.
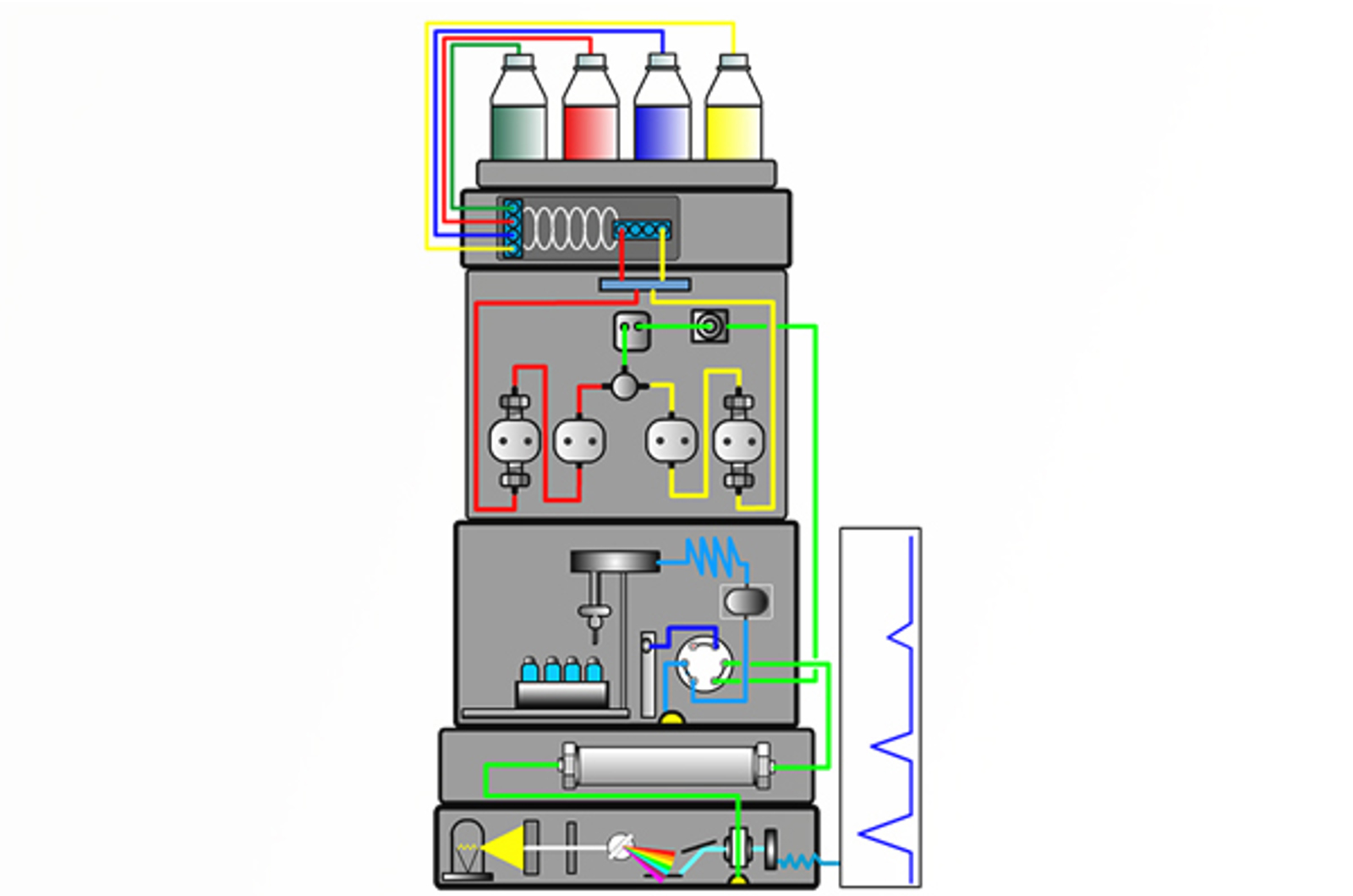
HPLC Introduction
The aim of this module is to give a brief history of liquid chromatography and compare and contrast high performance liquid chromatography (HPLC) with gas chromatography (GC). Liquid chromatography (LC) is explained, and the function of each component of the HPLC system discussed. The chromatogram is also introduced.
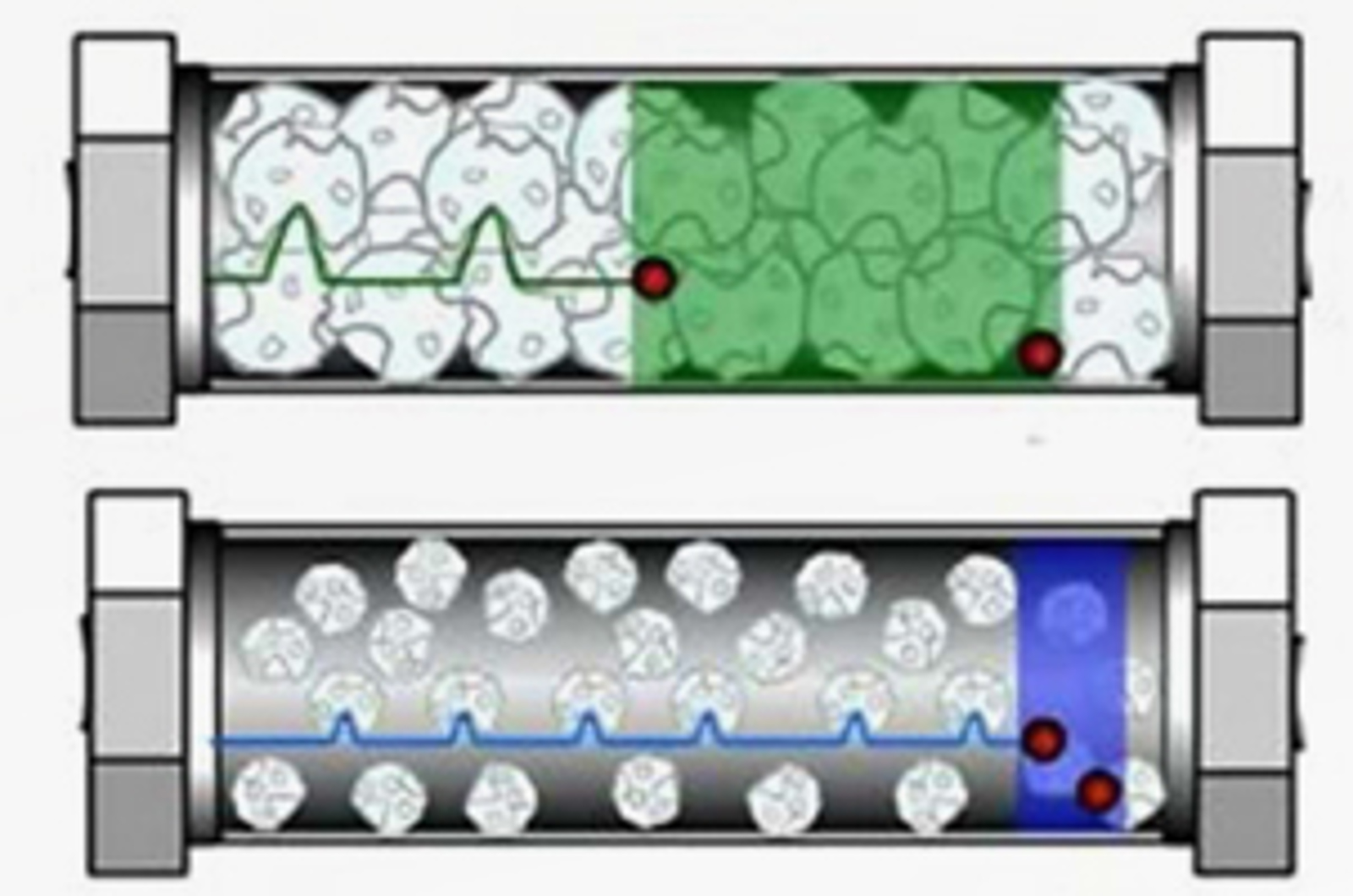
Core Shell Particles – Present and Future
In this session, our speakers highlight the benefits of core shell particle technology and the reasons behind their surprisingly high efficiency. They will also discuss where core shell particles fit against other efficiency improving measures such as the use of sub 2 μm particles with high pressures and also narrow bore HPLC columns.
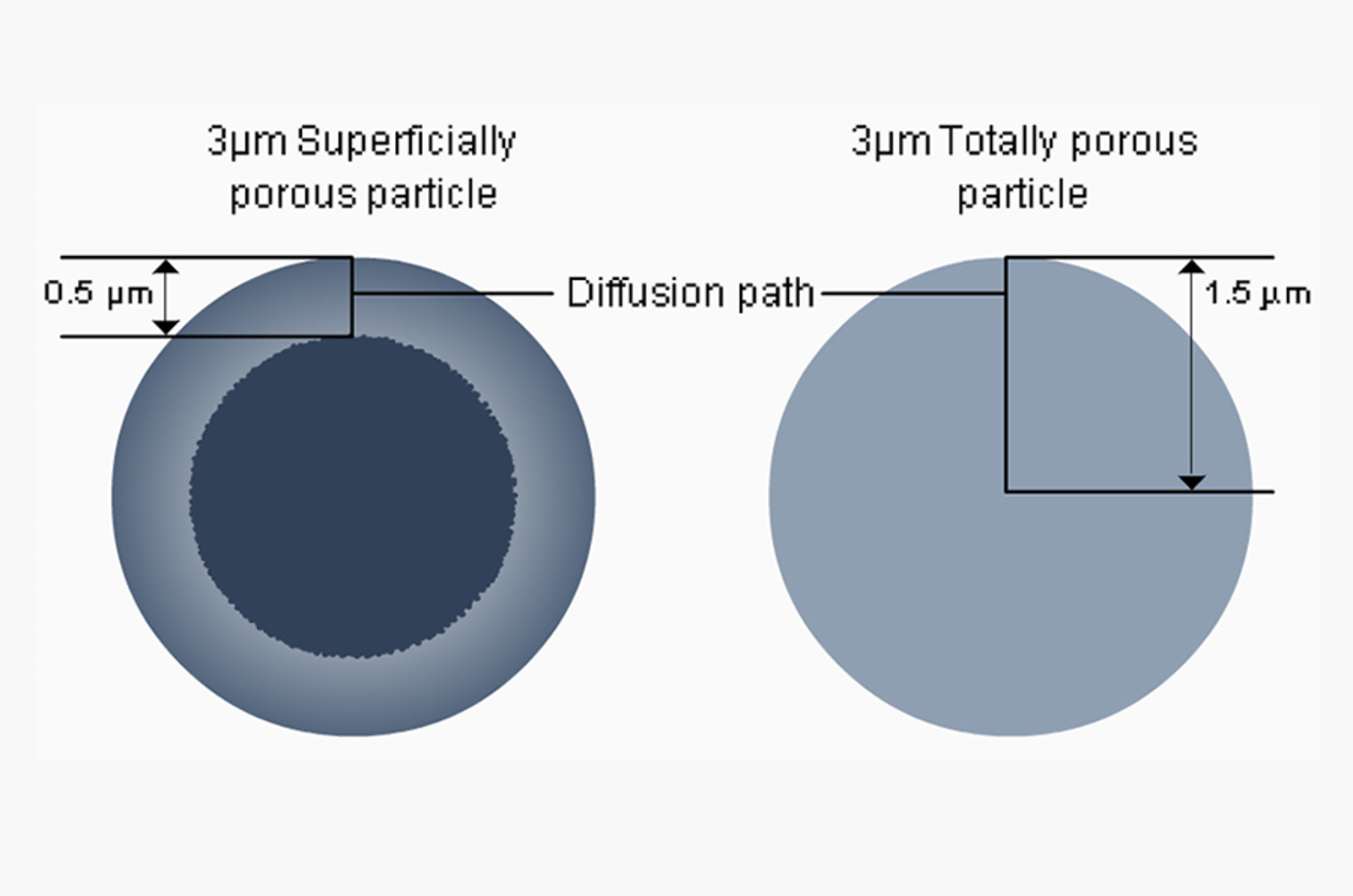
Superficially Porous or Sub 2 µm Stationary HPLC Phases
In this session, our speakers compare and contrast the relative advantages and disadvantages of employing HPLC columns packed with superficially porous or sub 2μm conventionally fully porous particles.
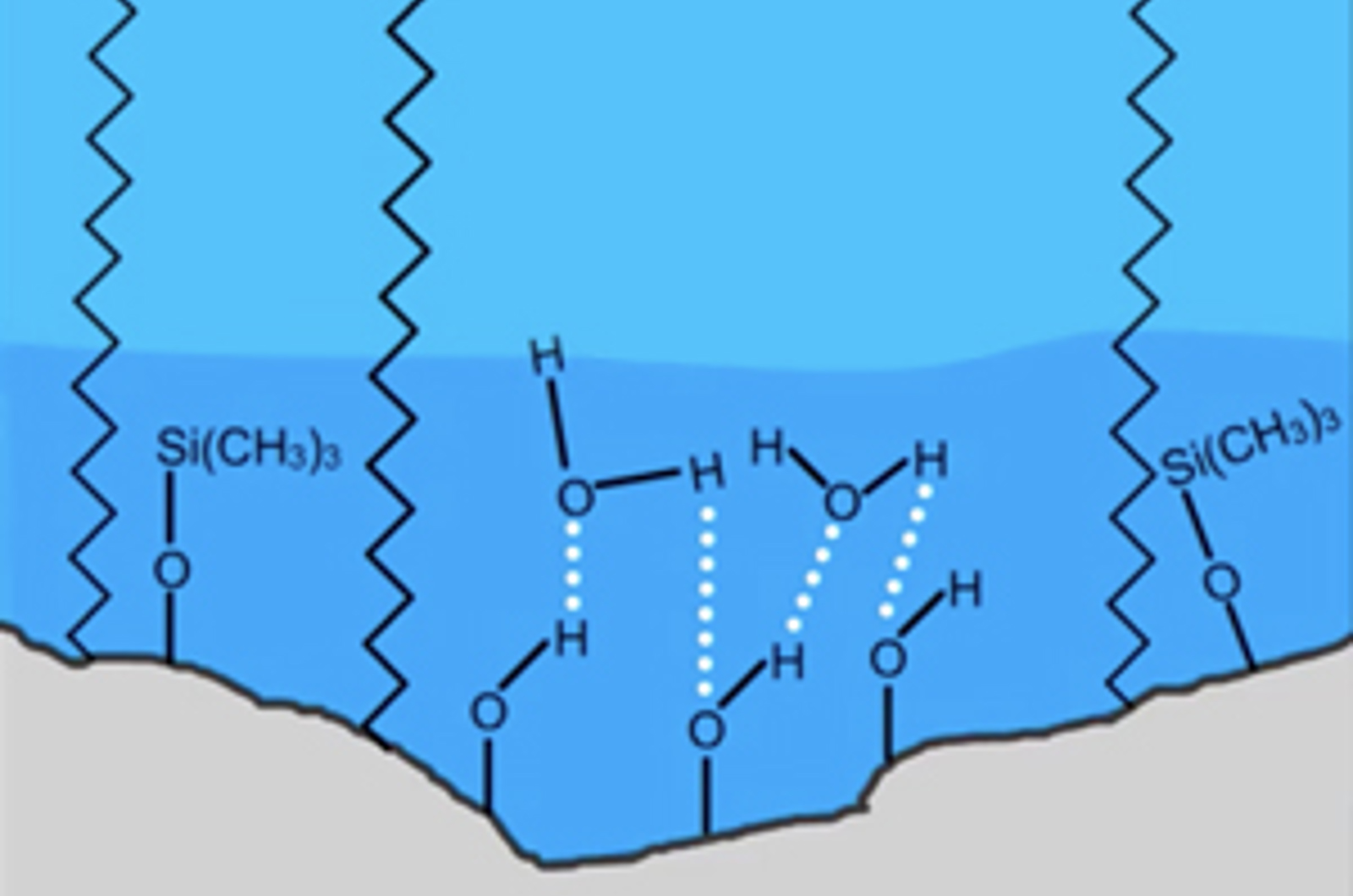
Understanding Stationary Phase Chemistry for Reversed Phase HPLC
In this webcast, our speakers brings you key information on choosing HPLC stationary phases for reversed phase and HILIC HPLC.
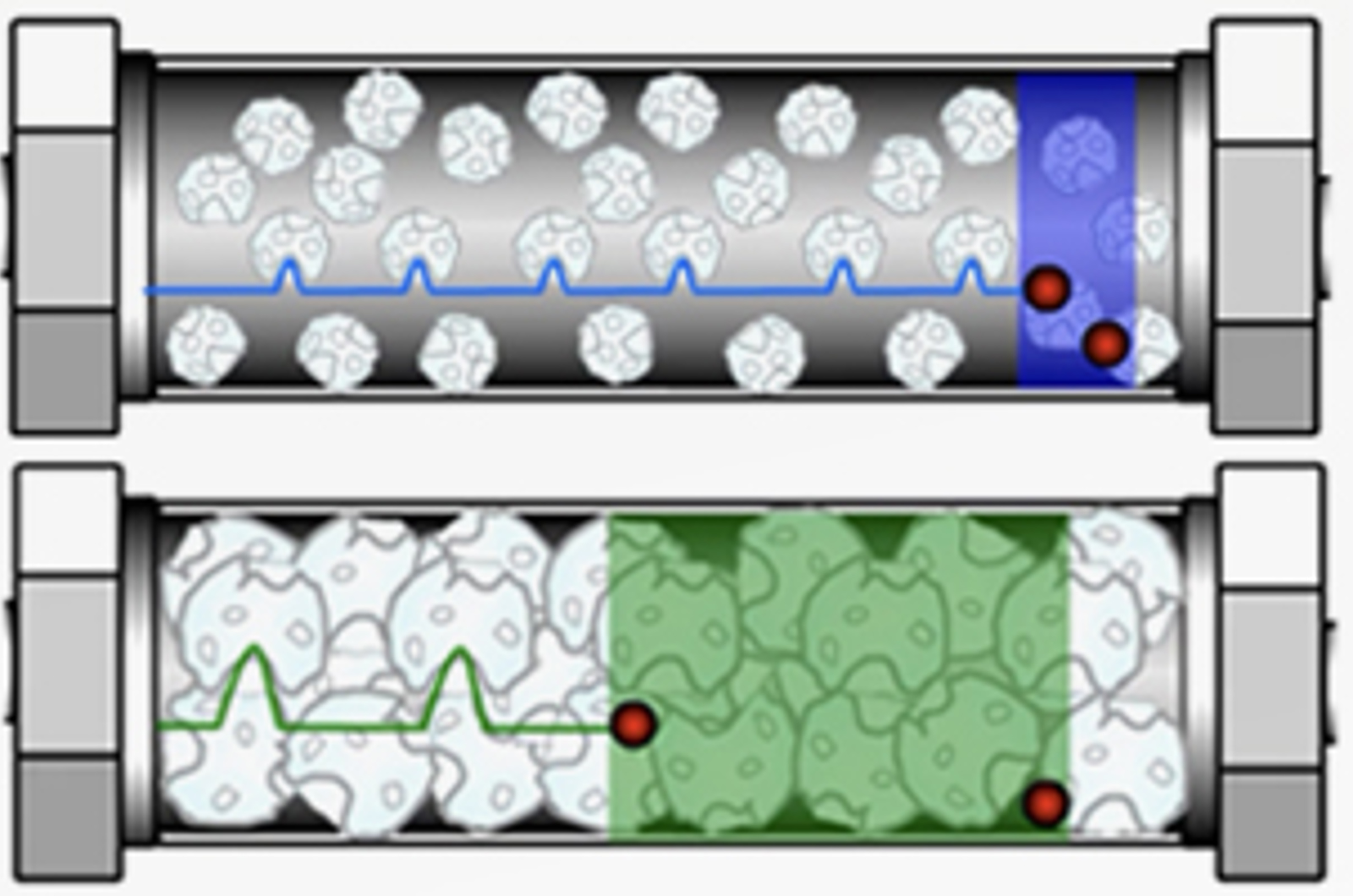
HPLC Band Broadening
The aim of this module is to illustrate the principle of band broadening in HPLC. The van Deemter equation and the terms of the equation will be defined and explained. The effects of eddy diffusion, longitudinal diffusion, and mass transfer on the efficiency of chromatographic peaks will be explored.
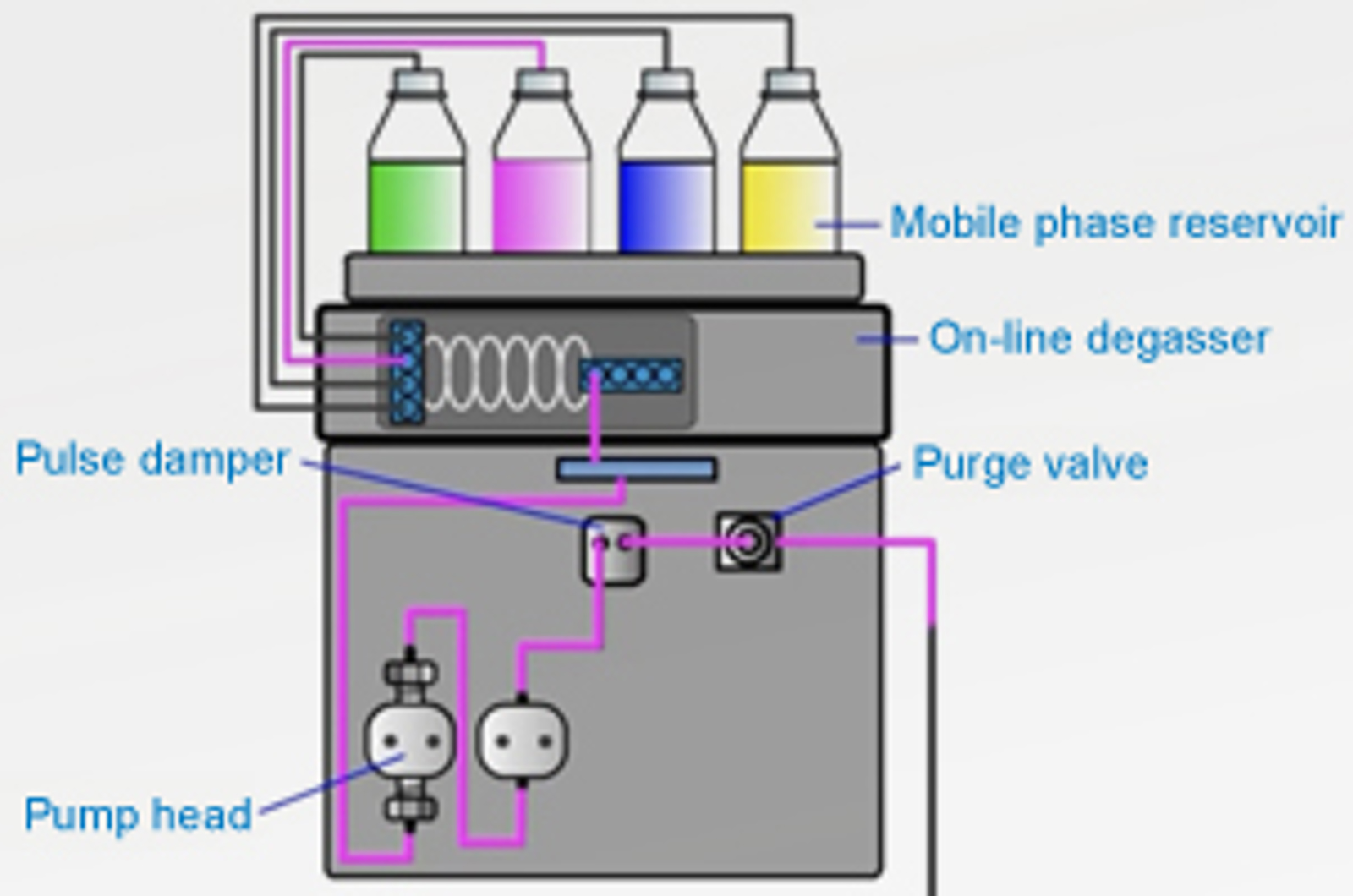
Mobile Phase Considerations
The aim of this module is to explain the purpose of the mobile phase in HPLC. Topics include the best way to prepare mobile phase, potential problems caused by improper preparation and use of mobile phases, and the way that mobile phase composition effects retention and selectivity in reverse phase HPLC.
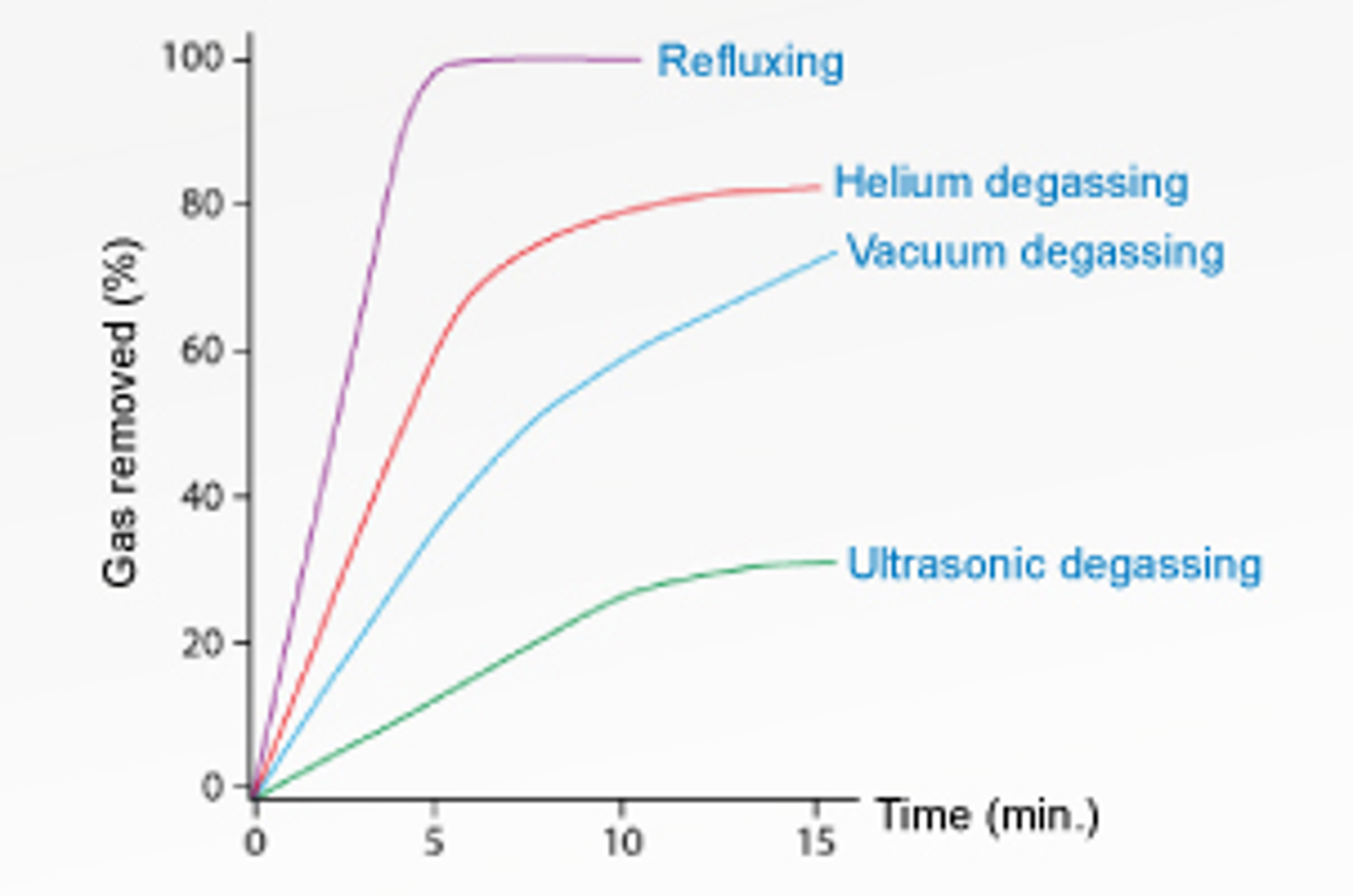
HPLC Mobile Phases – 10 Bad Habits to Avoid
Learn about the most common bad habits that should be avoided with HPLC mobile phases!
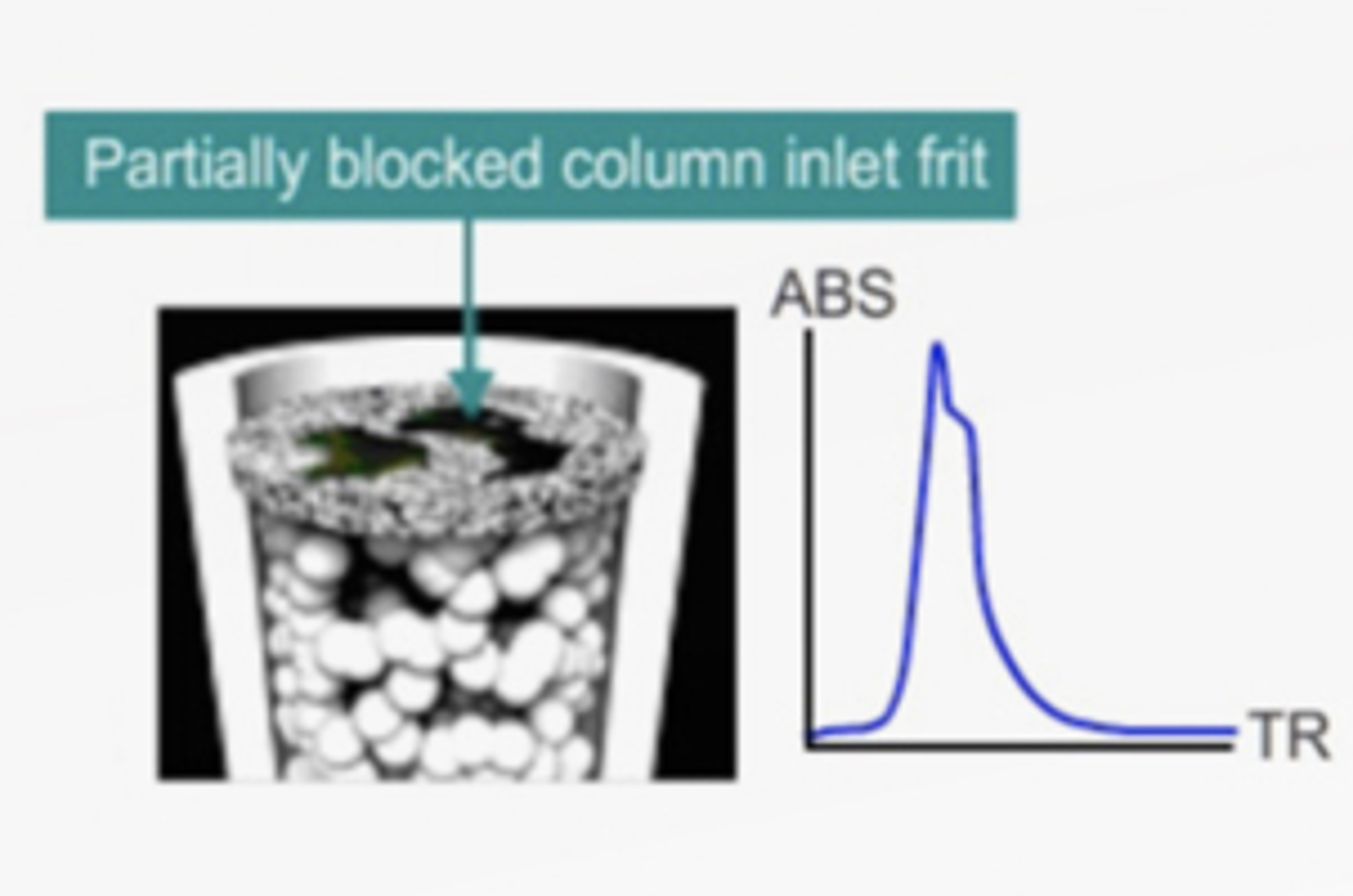
HPLC Column Abuse
Don’t let your HPLC column become just another statistic of abuse! Read these few short questions on HPLC column myths and be kind to your columns. They’ll appreciate it and work better for you.
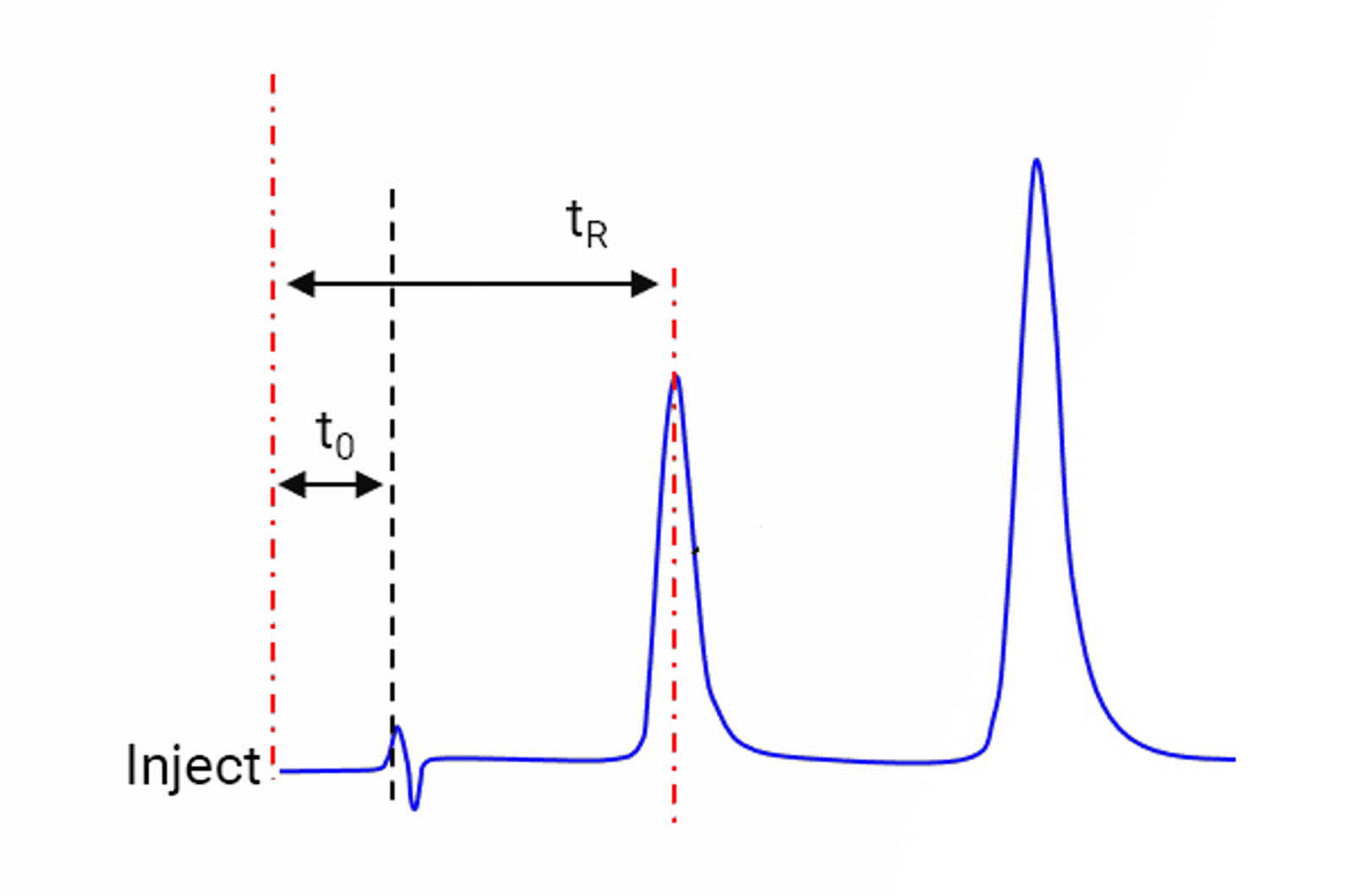
HPLC Column Dead Volume
Learn about HPLC column dead volume with this quick guide.
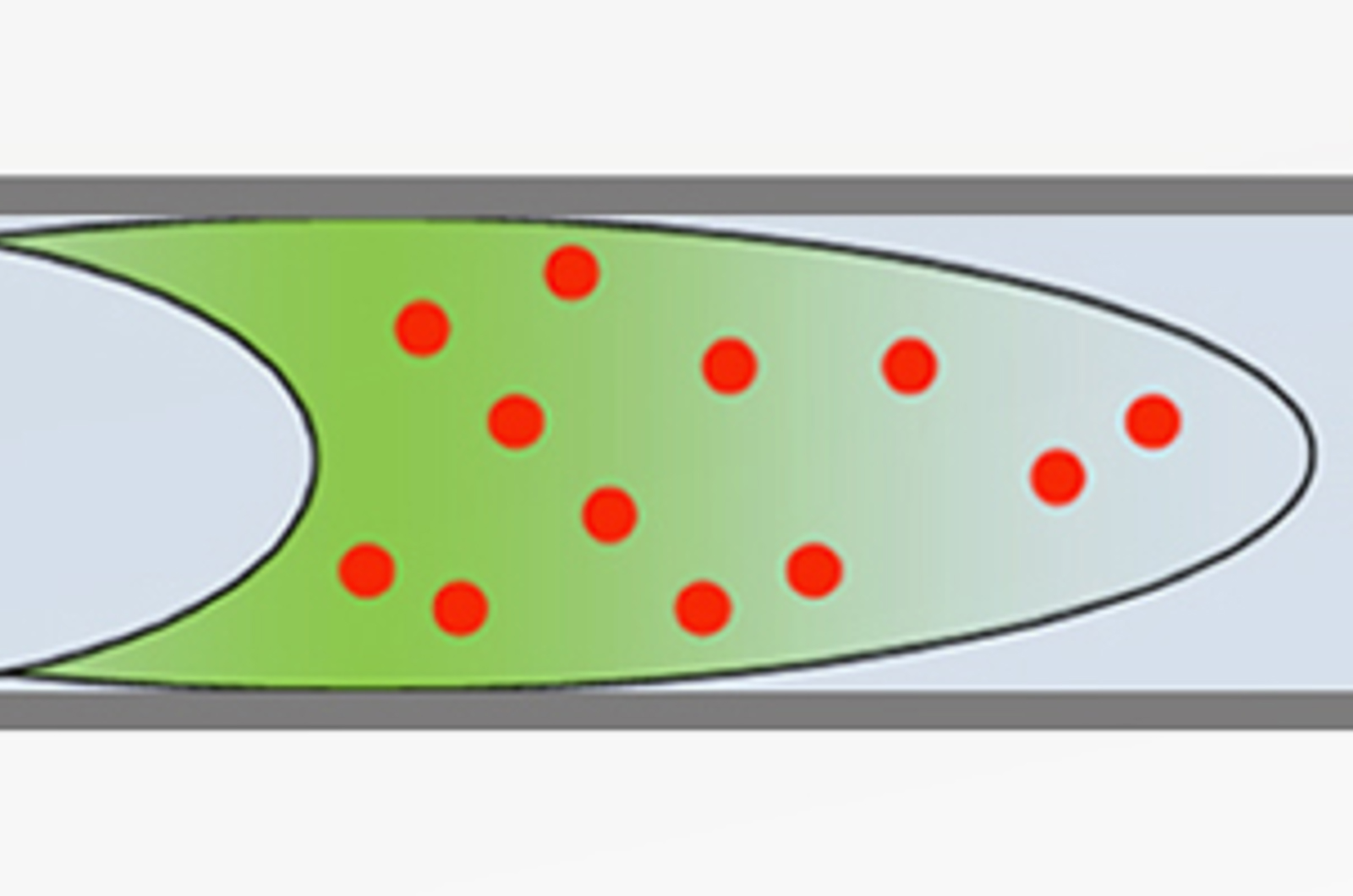
Sample Diluent Effects in HPLC
Most of us will know that the solvent (diluent) used to prepare HPLC samples can have an effect on HPLC peak shapes. The following discussion highlights some facts, figures, and tips and tricks that can help in a practical situation.
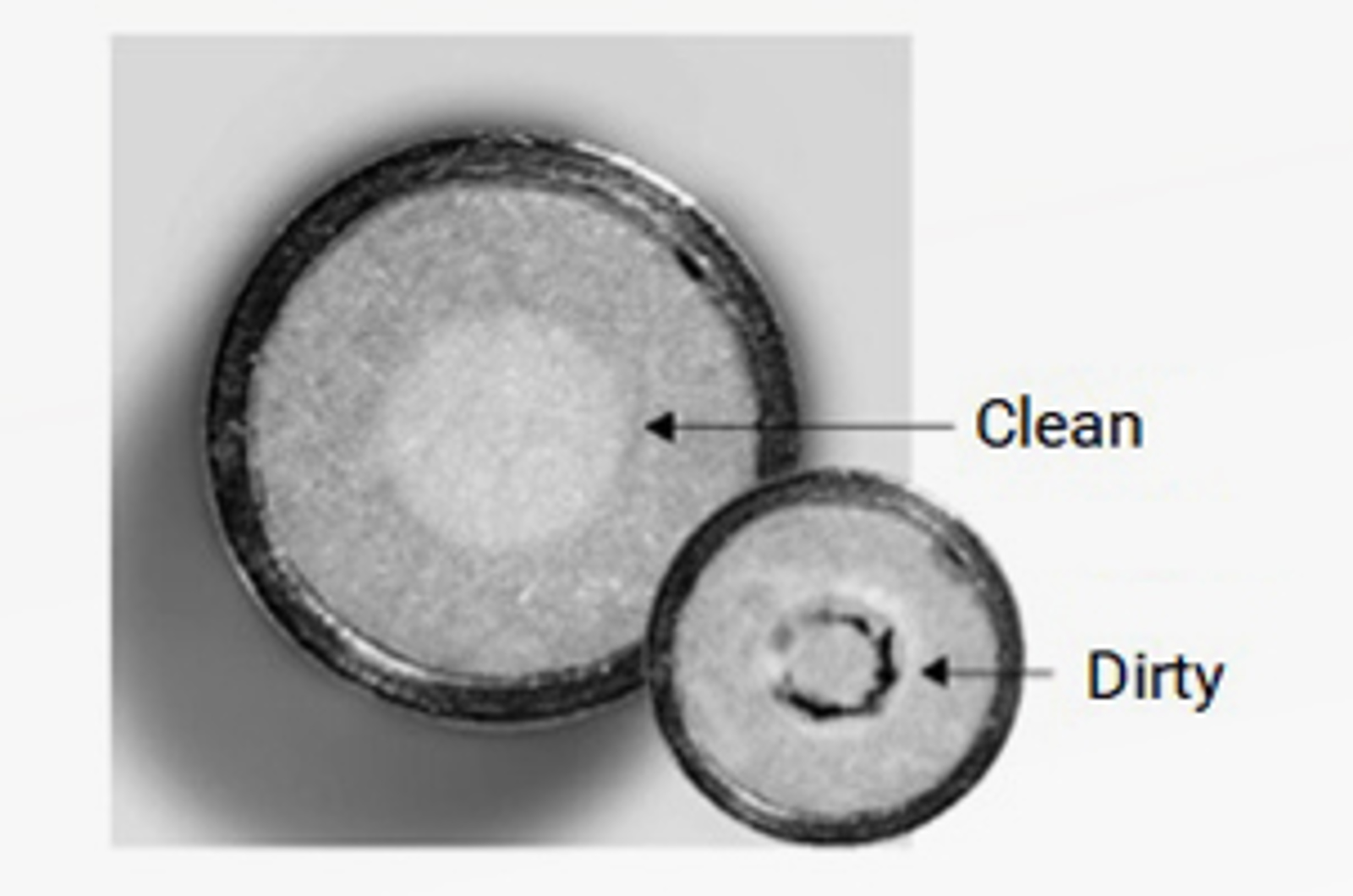
HPLC Column Maintenance - Prevention is Better than Cure
Preventing problems before they occur is always preferable to troubleshooting complex problems. This quick guide will look at practical steps that can be taken to ensure columns are well maintained so that they can perform as required, including dedicating columns, using in-line filters, guard columns or disks, utilizing sample clean up, proper column storage, and column replacement.
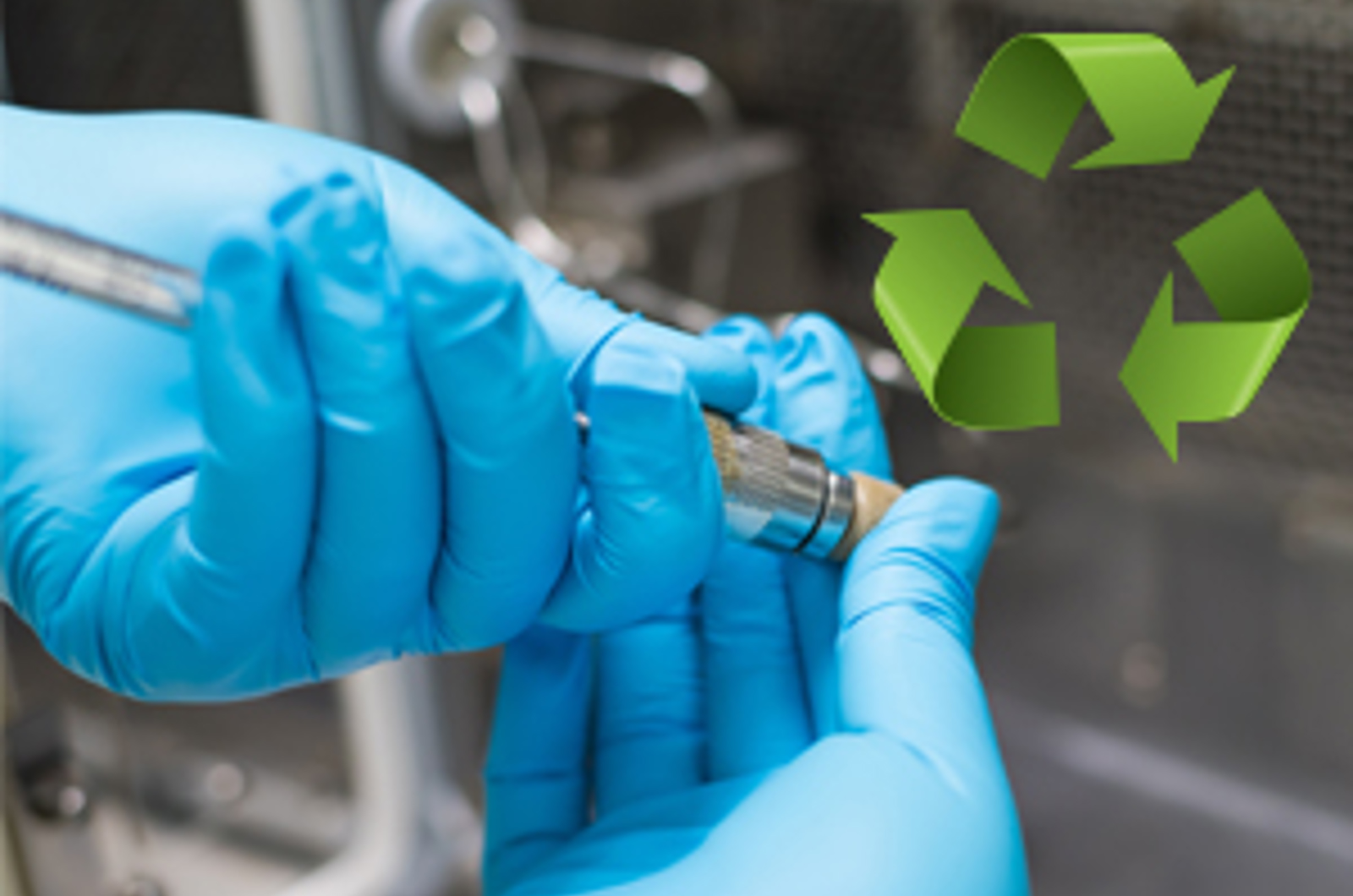
HPLC Column Cleaning and Regeneration
Column contamination from adsorption of analyte or matrix impurities at the head of the HPLC column can have deleterious chromatographic effects, such as, split peaks, changes in selectivity, and changes in quantitation. However, before you send your column to the scrap heap it can be worth trying to clean and regenerate it!
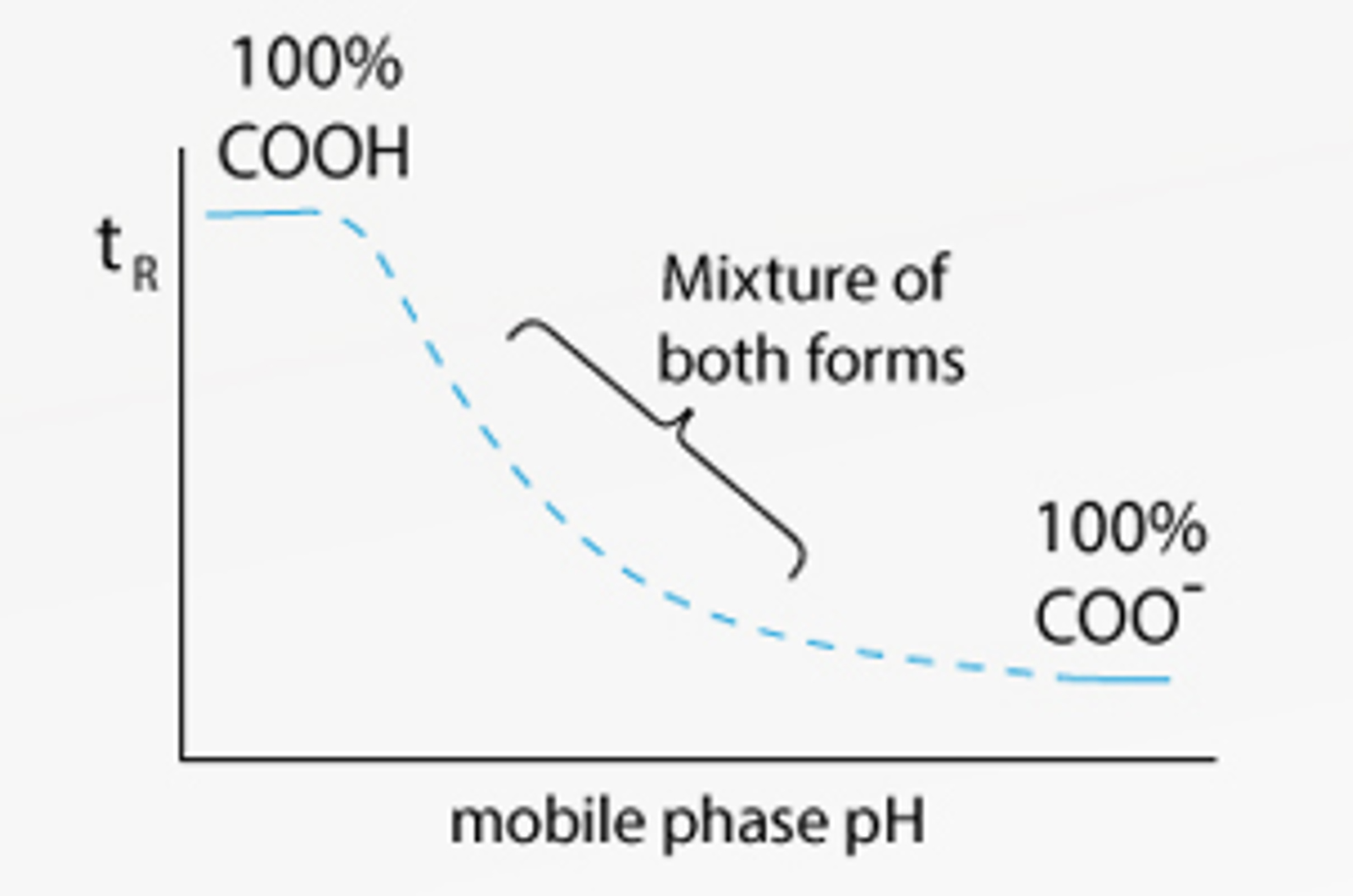
Why is pH Important for HPLC Buffers?
Learn why pH is important for HPLC buffers in this quick guide.
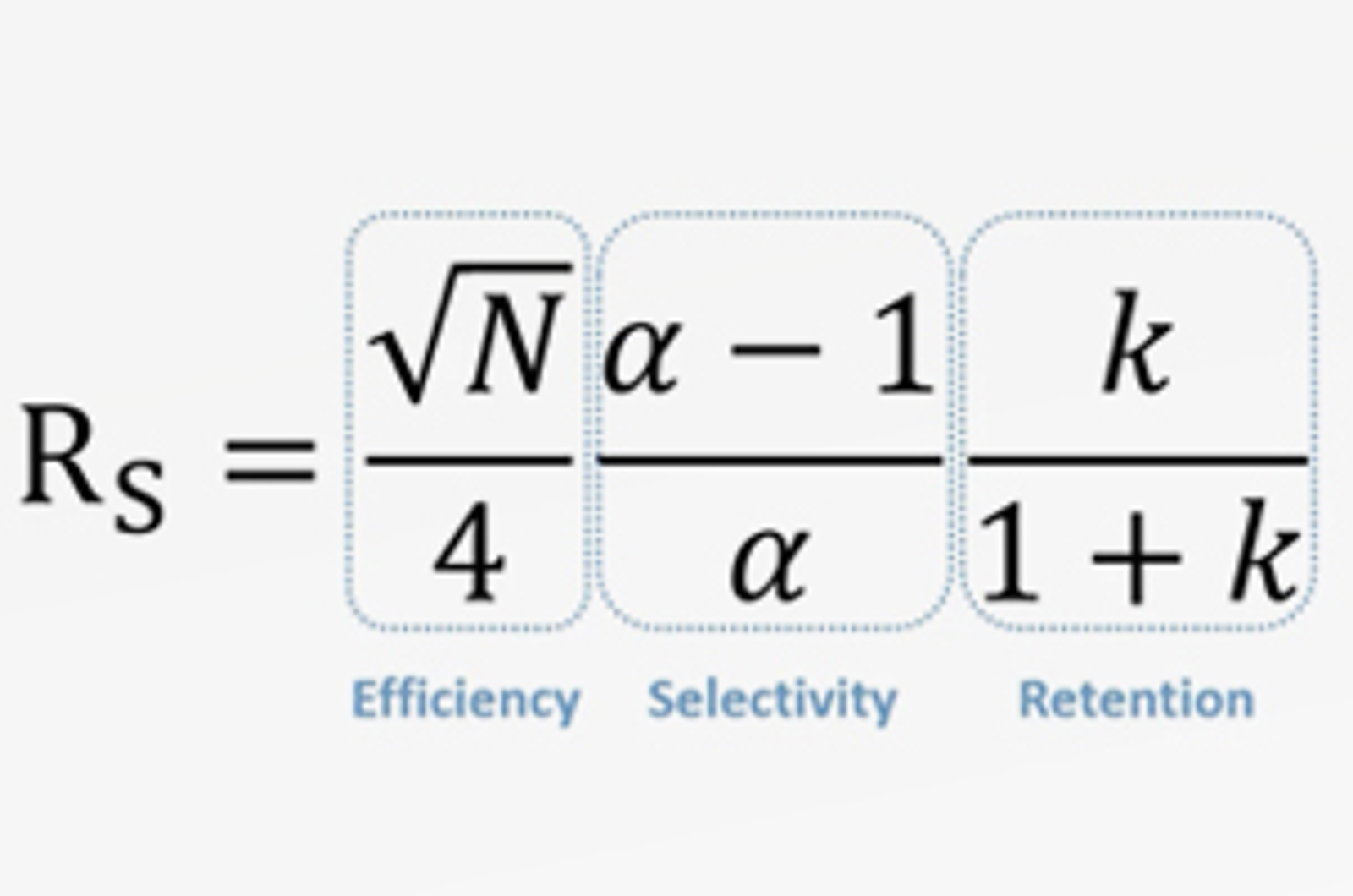
HPLC Chromatographic Parameters - Retention Factor
The aim of any HPLC method is to provide optimum resolution of your analytes in the shortest amount of time. Resolution is governed by three chromatographic parameters, efficiency, selectivity, and retention. This short video will discuss retention factor in more detail, looking at what it describes in terms of your separation and chromatogram, how it can be changed, and what effect it can have on the resolution of a separation.
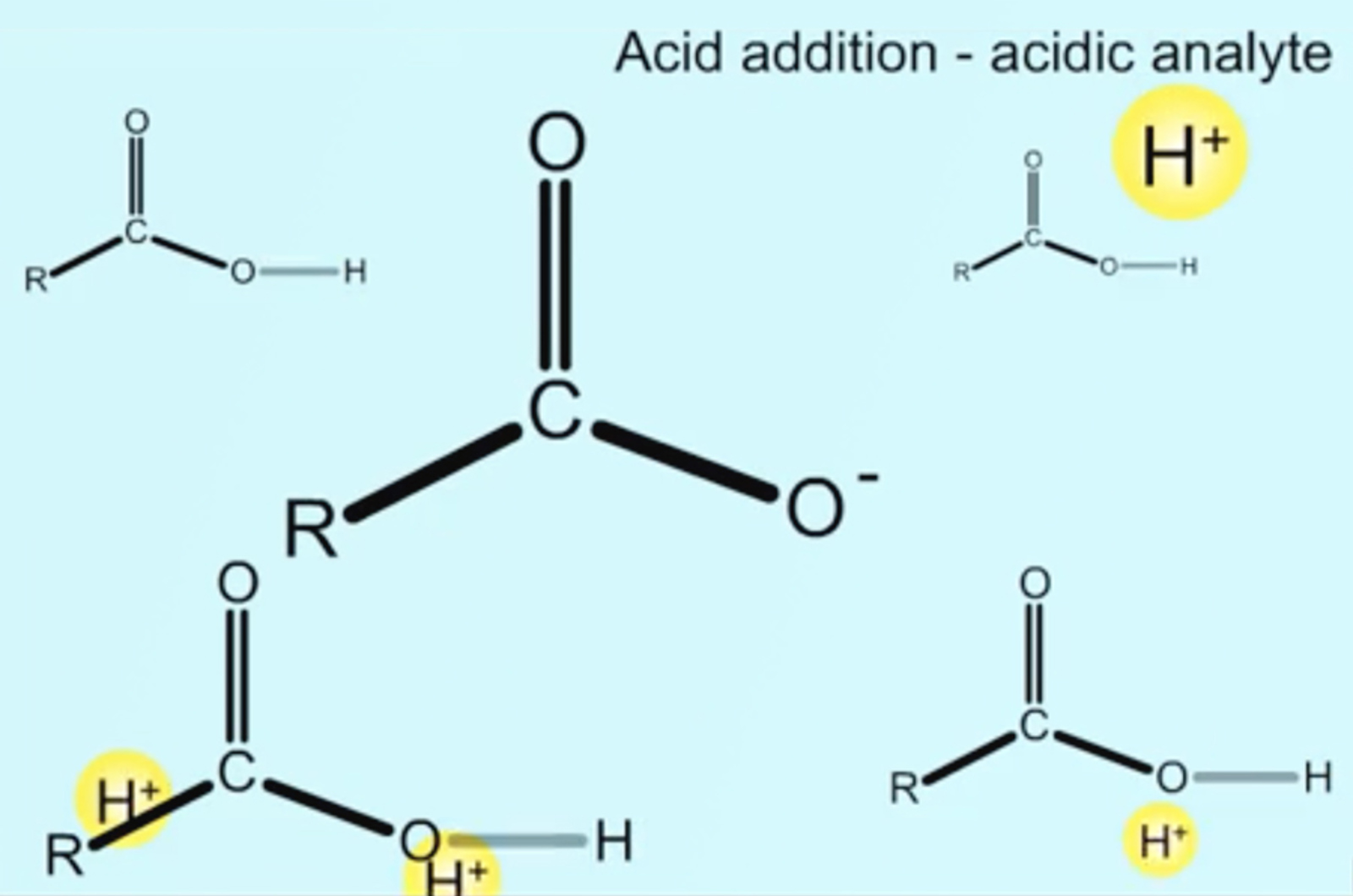
HPLC - Controlling Ionization
In reversed phase HPLC ionizable analytes present their own particular separation challenges and opportunities. When working with ionizable analytes, it is important to consider the pH of the mobile phase to optimize separation, that is to alter and optimize retention, selectivity, and efficiency. This short video will consider how analyte ionization state can be manipulated to achieve the required chromatographic separation, and which method parameters are used to do this.
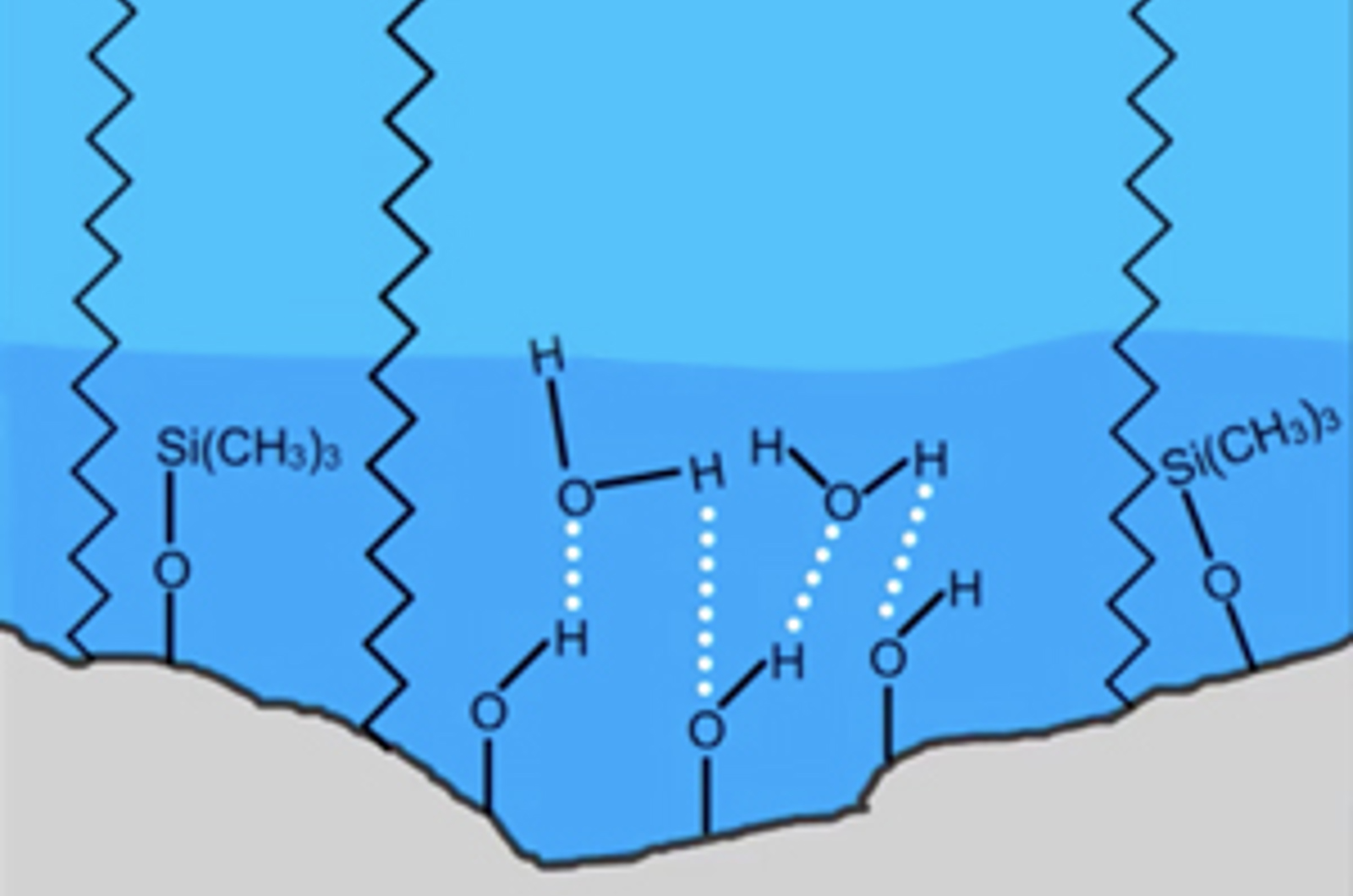
HPLC Columns – Water Wettable Phases
Column selection is possibly one of the most important steps in HPLC method development. The chemistry of both the analytes and stationary phase need to be considered as well as any requirements of the detection process (e.g. ionization state for LC-MS). In this short video, we will look at columns which are designed for challenging applications which require the use with highly aqueous mobile phases.
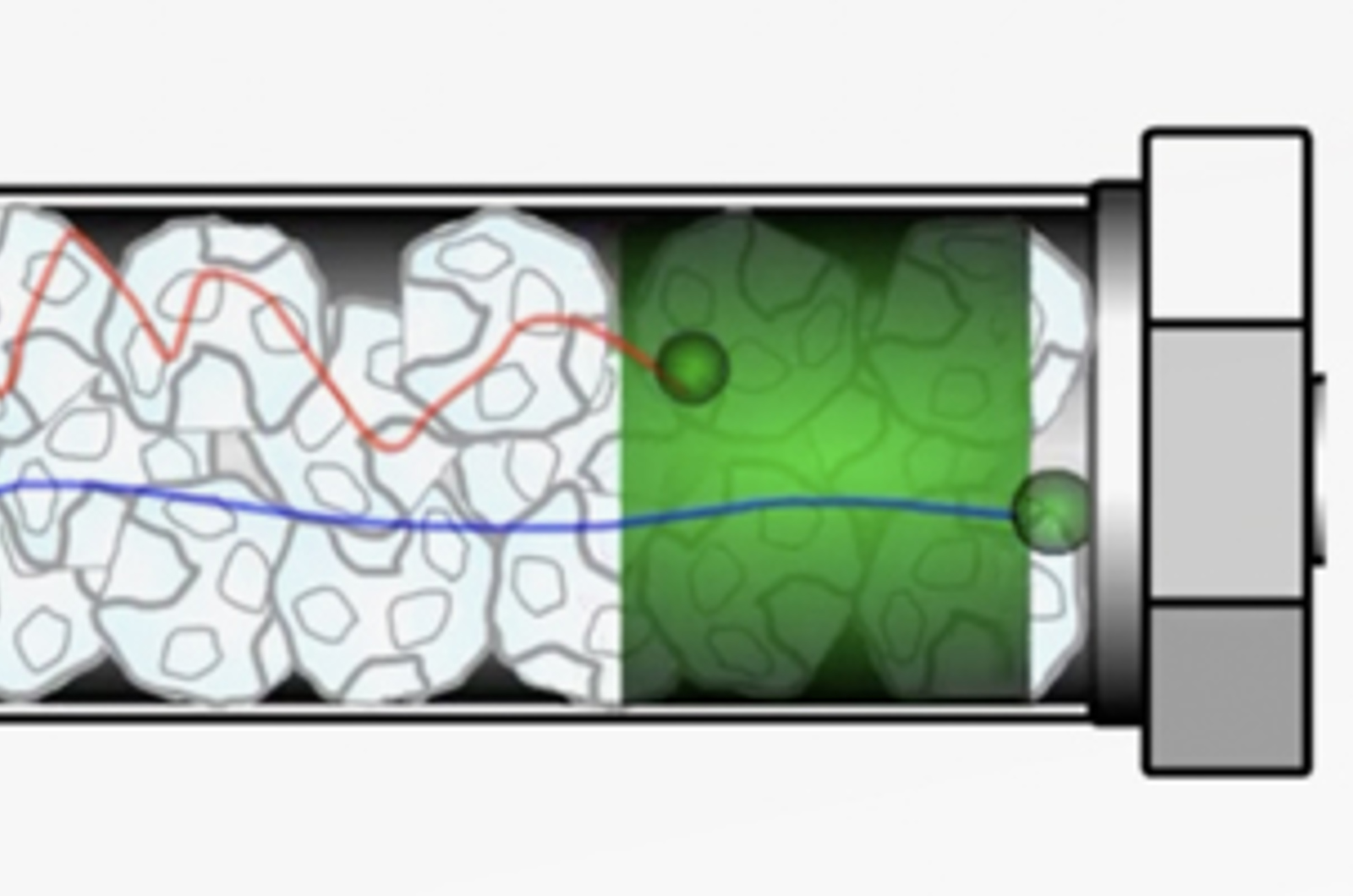
HPLC Band Broadening - Eddy Diffusion
Band broadening is a phenomenon that reduces the efficiency of the separation being carried out; leading to poor resolution and chromatographic performance. There are several factors which contribute to band broadening all of which should be understood to minimize their effects. This video considers eddy diffusion, how it impacts chromatography, and how its contribution can be minimized.
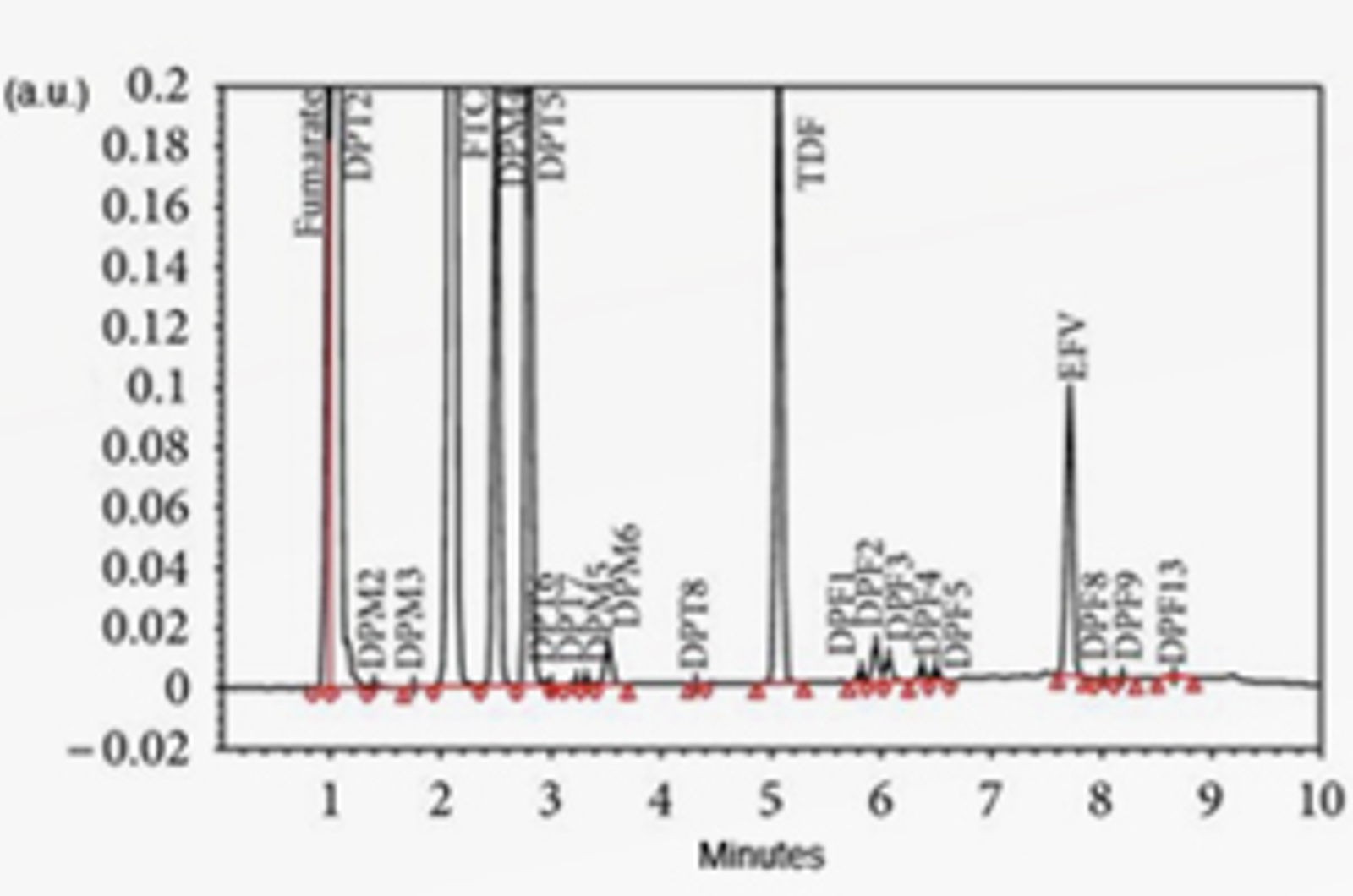
Setting HPLC Chromatographic Parameters
Whether you work in a regulated environment or not, setting chromatographic performance targets can help to keep us focussed. Let’s consider how to set these targets and examine some real life examples that may not always follow the rules.

Fundamentals of HPLC Video Training Course
This course has been designed for novice HPLC users and those who have some experience of running HPLC methods.
Endorsed by
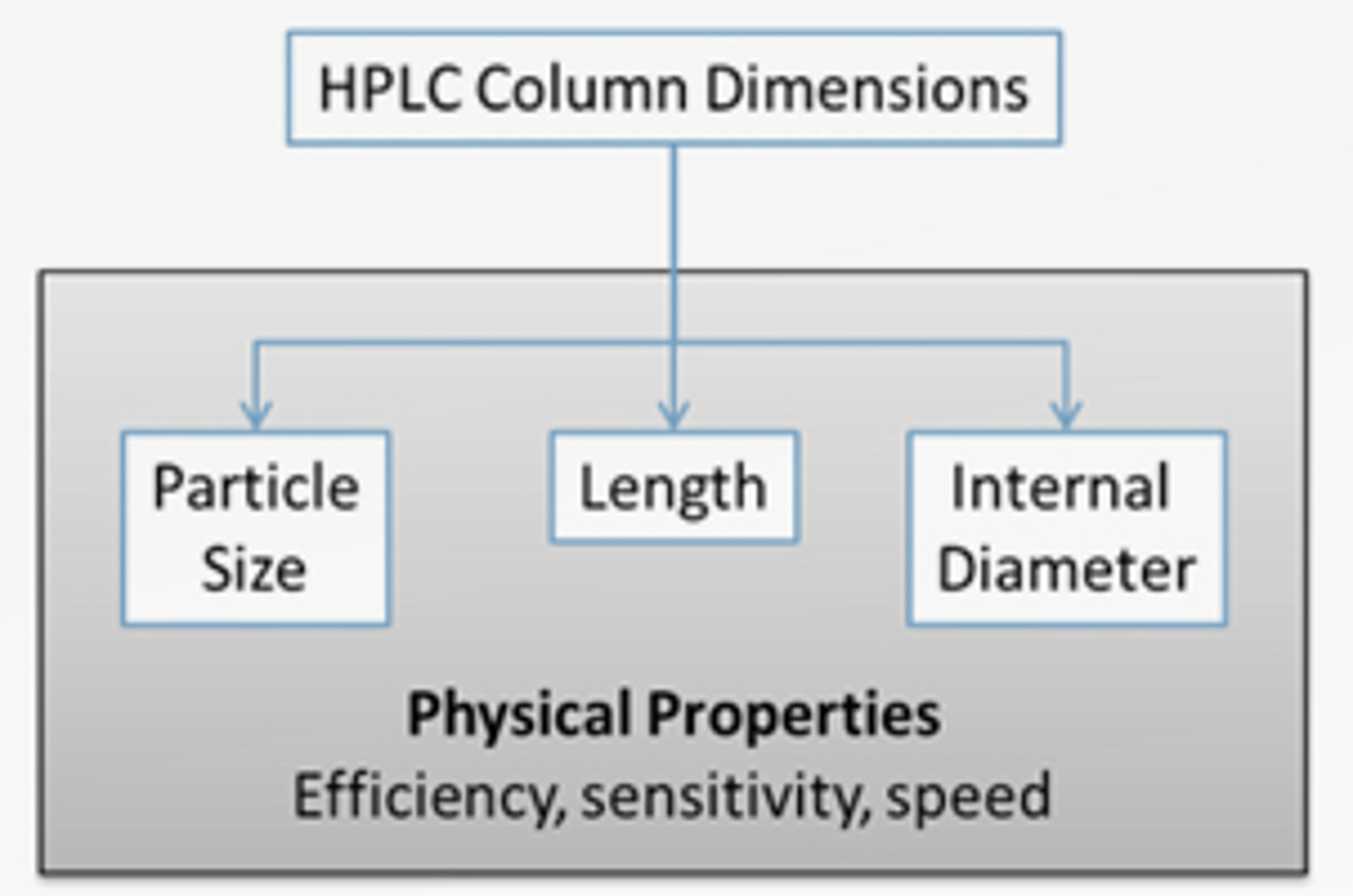
HPLC Column Dimensions
HPLC column dimensions will affect the efficiency sensitivity, and speed of an analysis. The primary column dimensions of particle size, length, and internal diameter will be discussed and their effect on chromatographic separations demonstrated, providing some practical insight into how to select the best column dimensions for a particular application.
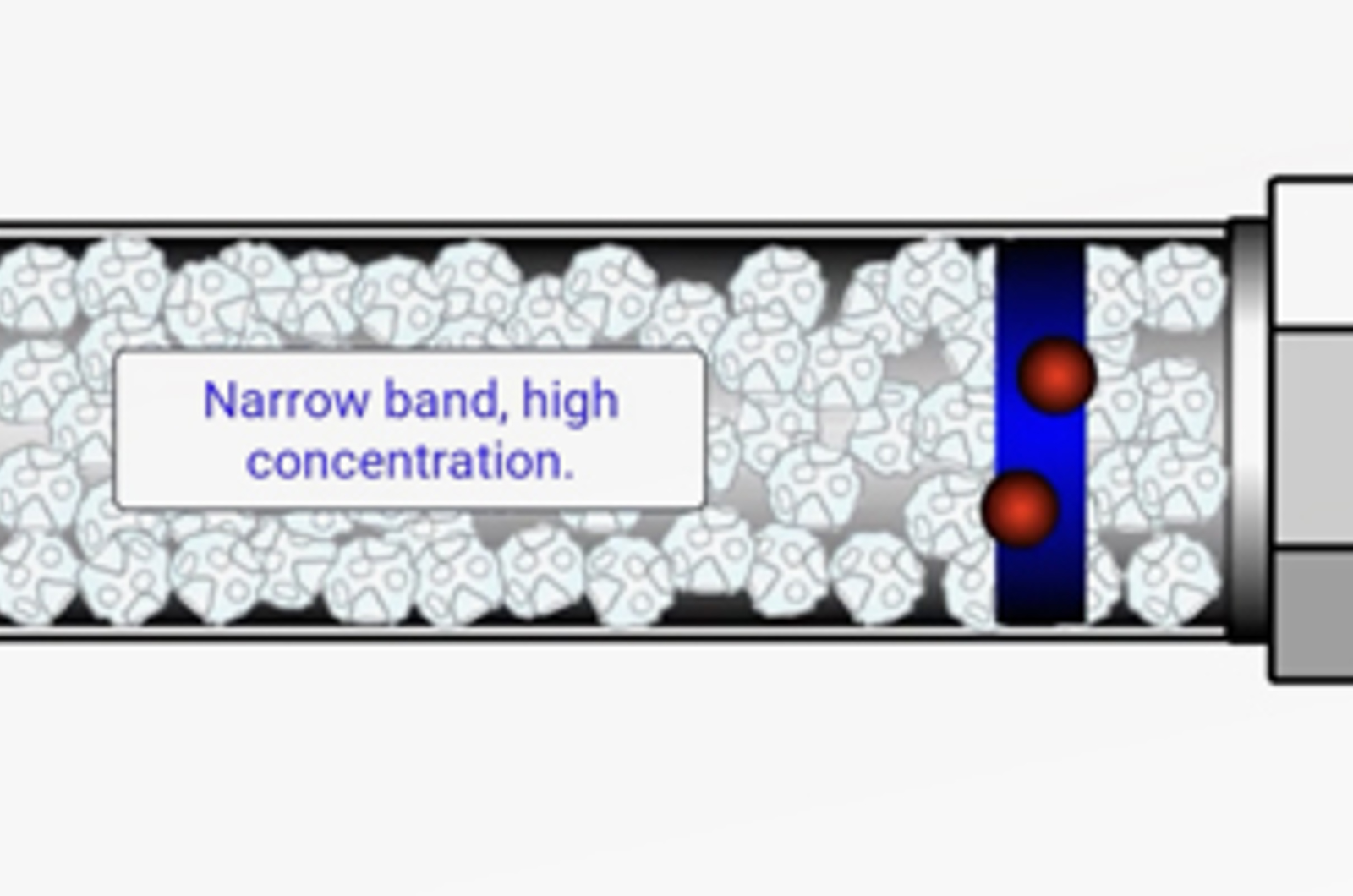
HPLC Extra Column Volumes
It is of particular importance to reduce extra column volumes when using small volume columns or UHPLC. However, where do these extra column volumes come from, how can they be minimized, and what effect do they have on chromatography?
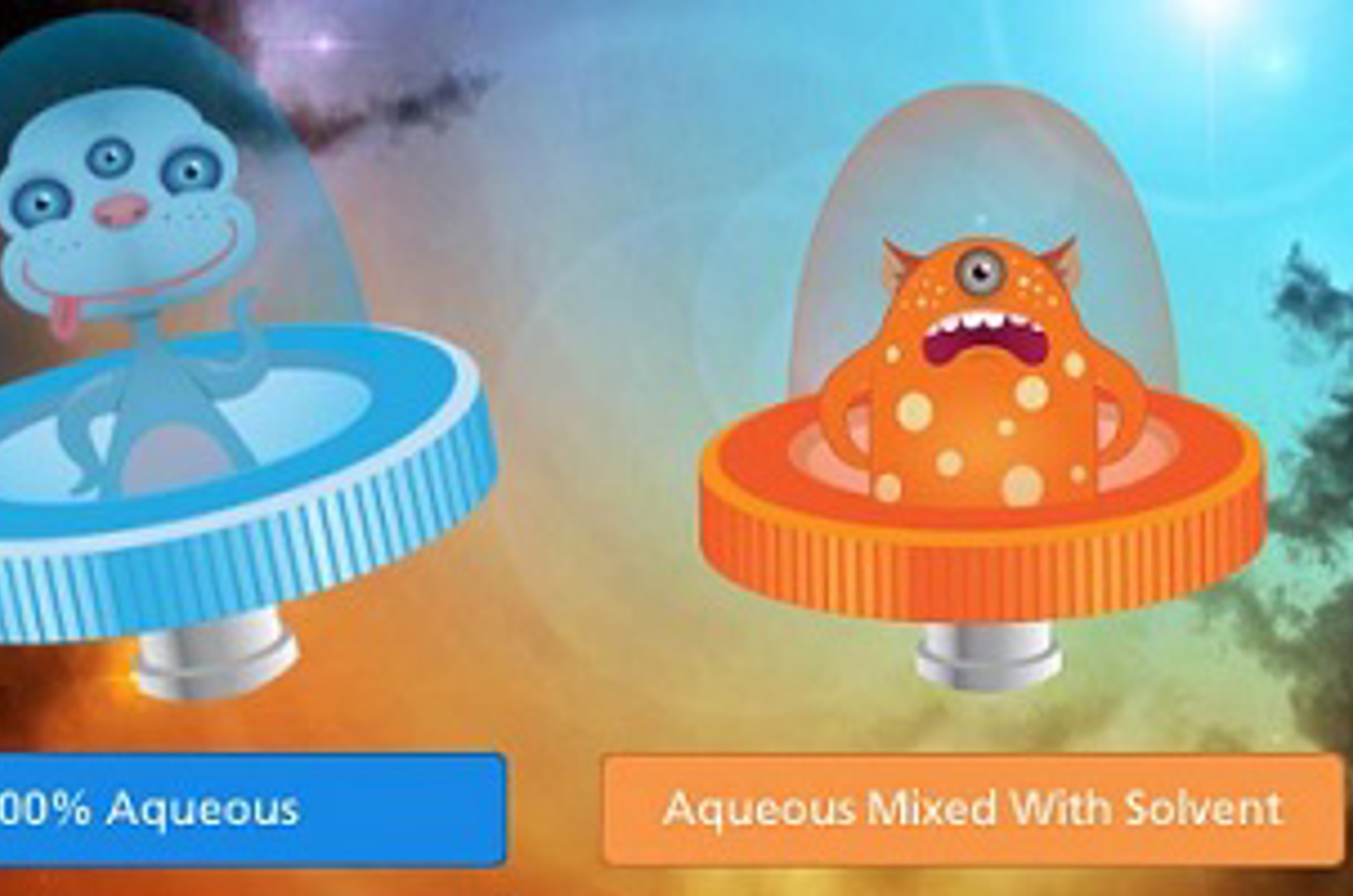
HPLC Filtration - Rocks, Boulders, and Sand
We get asked a lot about the value of mobile phase filtration and, especially since the introduction of UHPLC, the various system filters and how best to protect HPLC systems to allow maximum up time. We have collected together an assembly of thoughts on all things filtration for HPLC – and how to deal with various nasties – from rocks to sand.
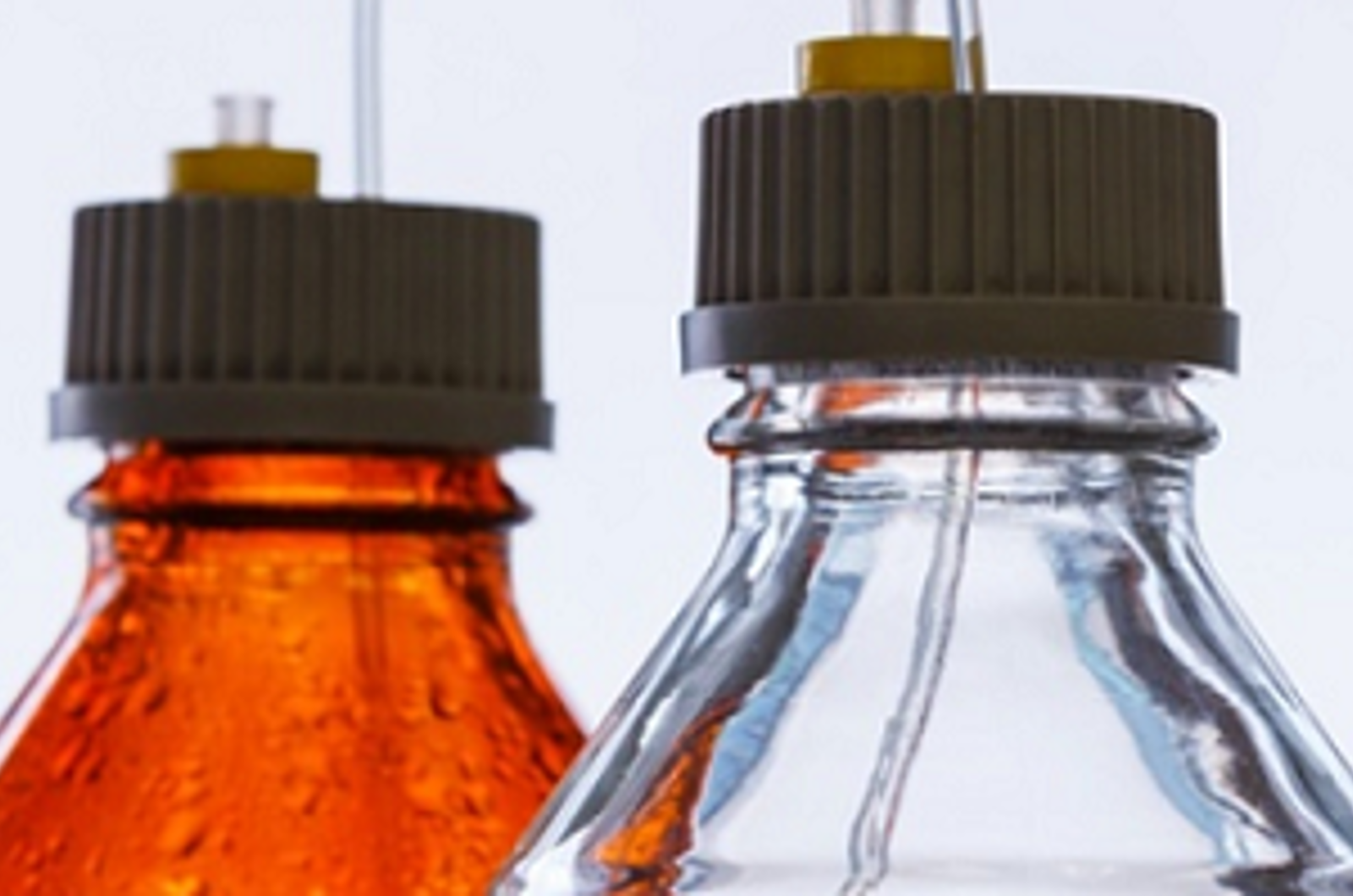
20 Steps to a Reliable HPLC Mobile Phase
Selecting a mobile phase and correct preparation are essential for a robust HPLC method. This webcast provides a fail safe 20 step guide to preparing the optimum mobile phase. This includes controlling pH, eluent mixing, filtration and degassing, mobile phase shelf life, and some of the most common mistakes to avoid.
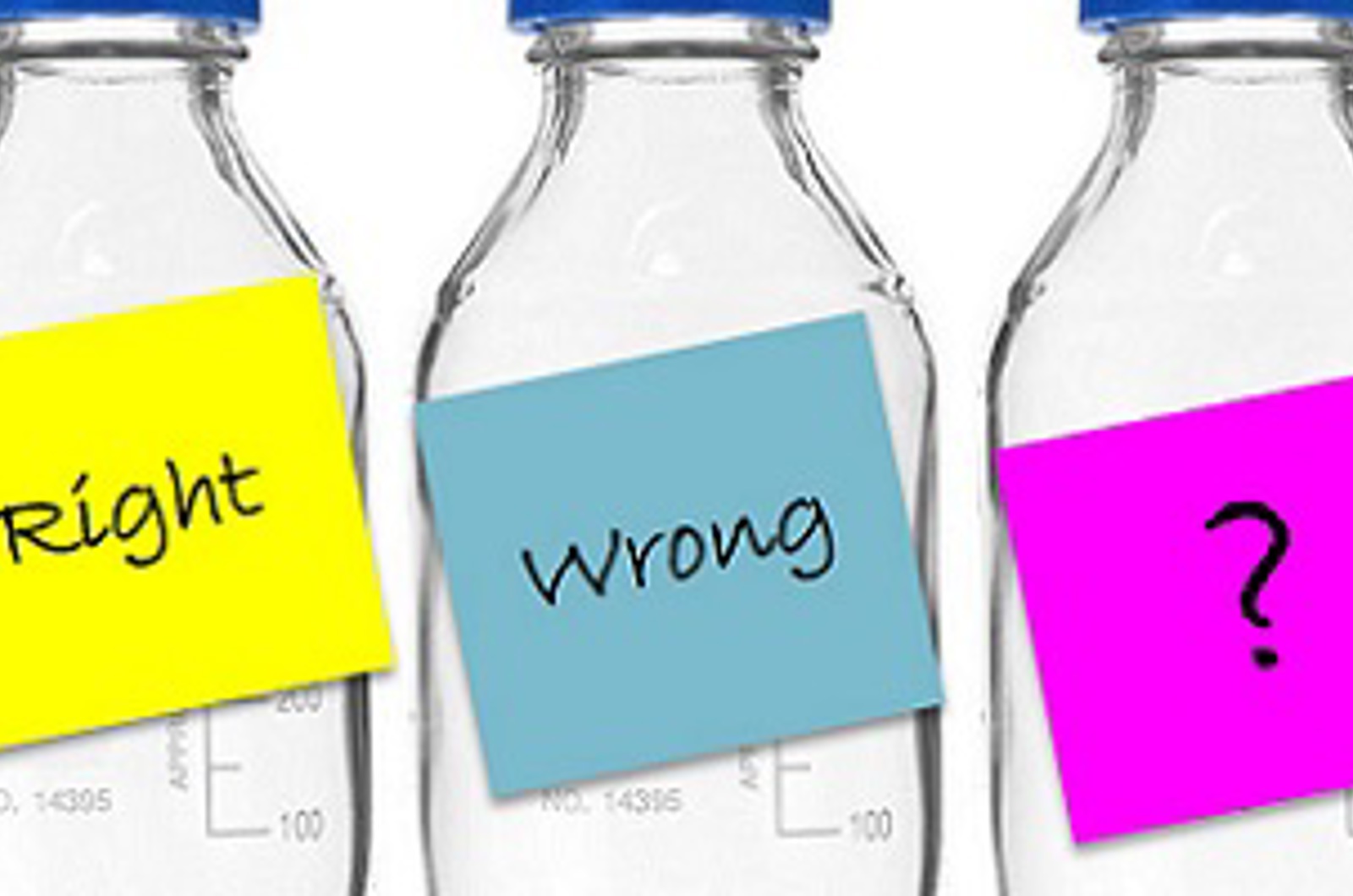
The Rights and Wrongs of Mobile Phase Preparation
Mobile phase preparation - what could possibly go wrong? Let us walk you through a typical mobile phase preparation process highlighting how to avoid common pitfalls such as contamination from glassware or mixing organic and aqueous in the incorrect order. After watching this webcast there will be much more right than wrong with your mobile phase preparation.
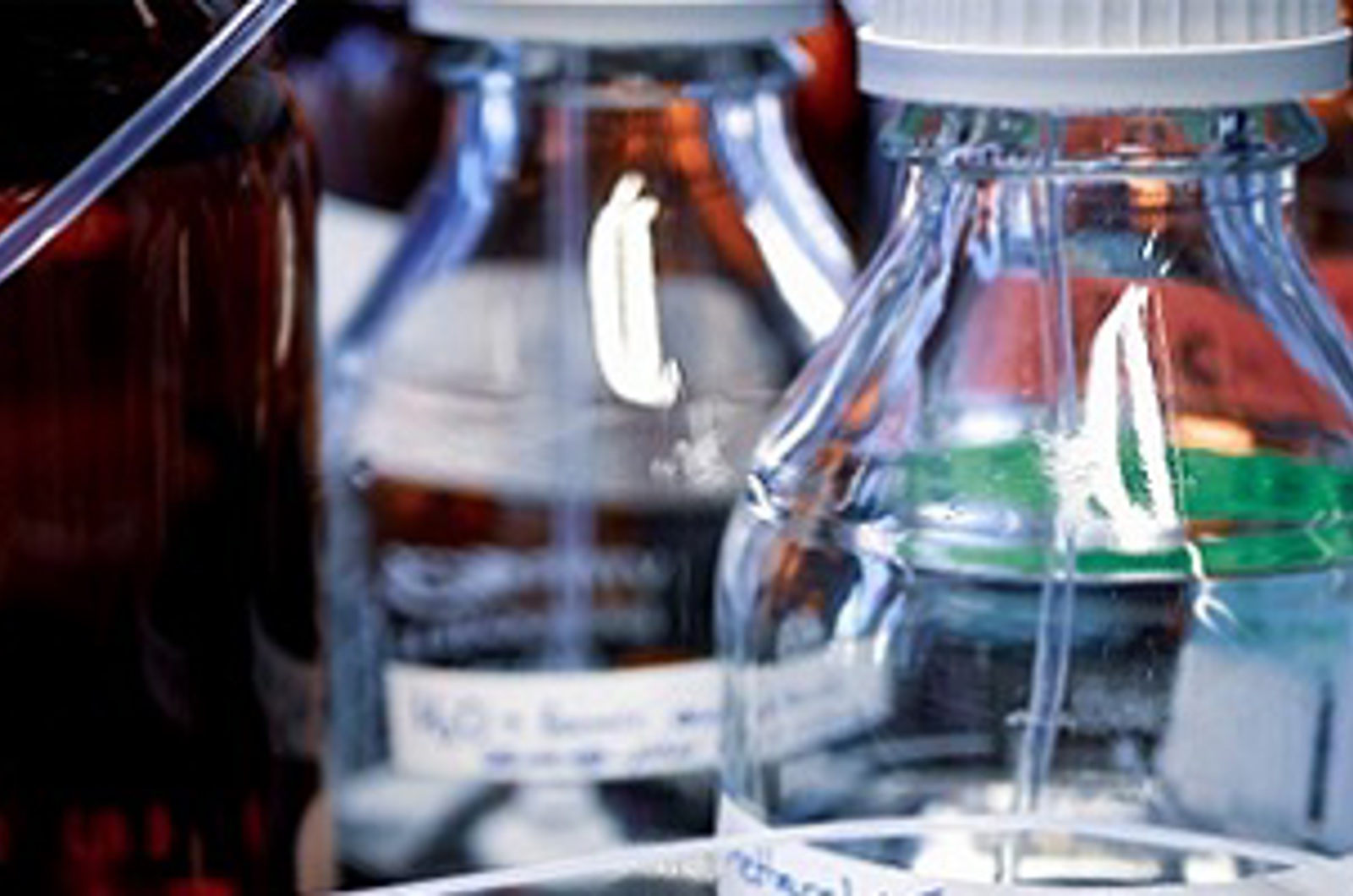
The Rights and Wrongs of Mobile Phase Preparation Q&A
This Q&A session, based on the questions submitted in one of our most attended webcasts, will make you think about mobile phase preparation in a whole new way! Everything from how cleaning our glassware can impact analyses, to how to avoid or minimize contamination, what the best practices are for filtering mobile phases - and lots in between.
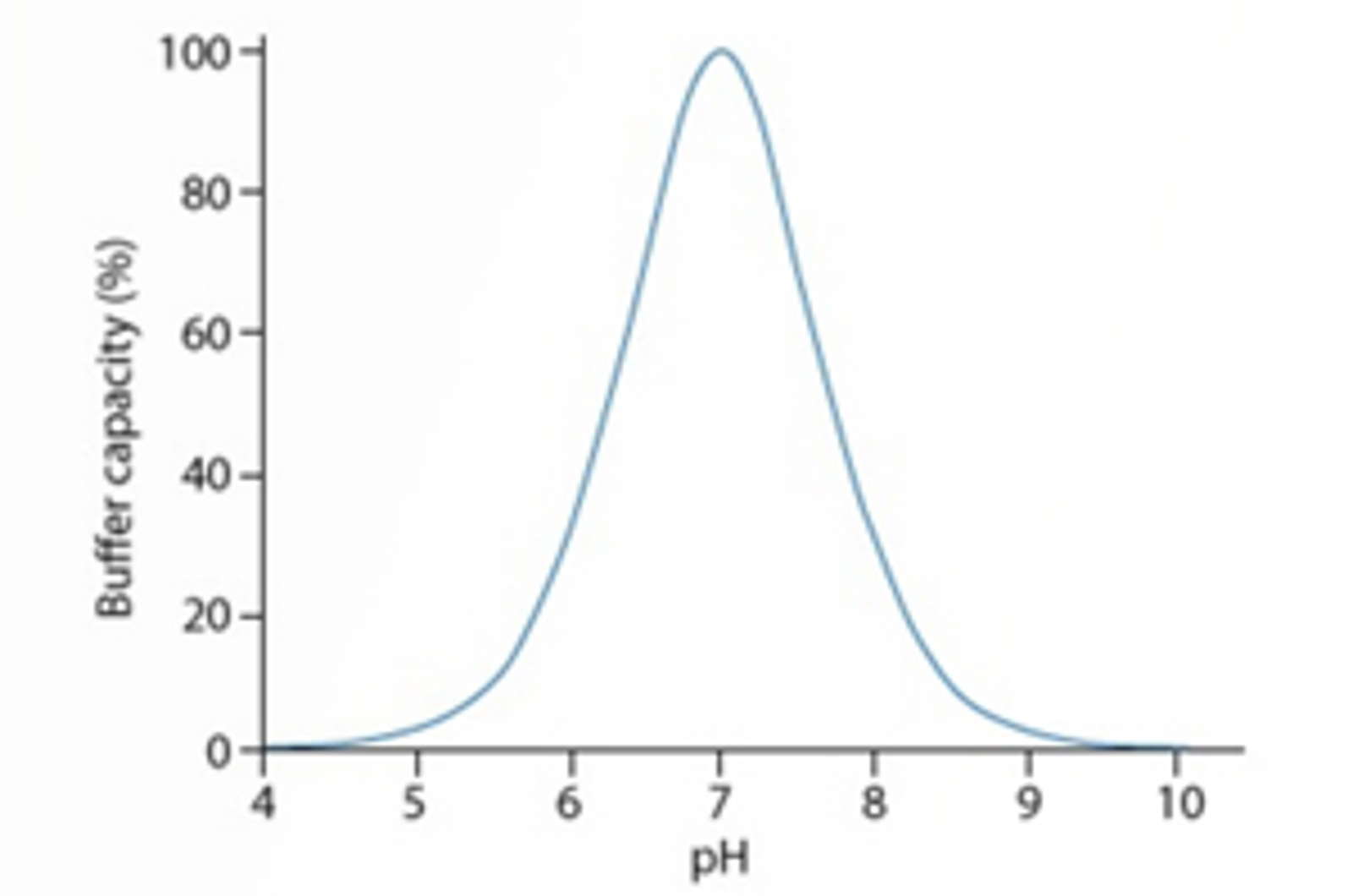
Buffer Choice for HPLC Separations
This quick guide looks at the important topic of buffer choice for HPLC separations; how to the choose the correct buffer type and concentration, as well as how to avoid variability in retention and selectivity.
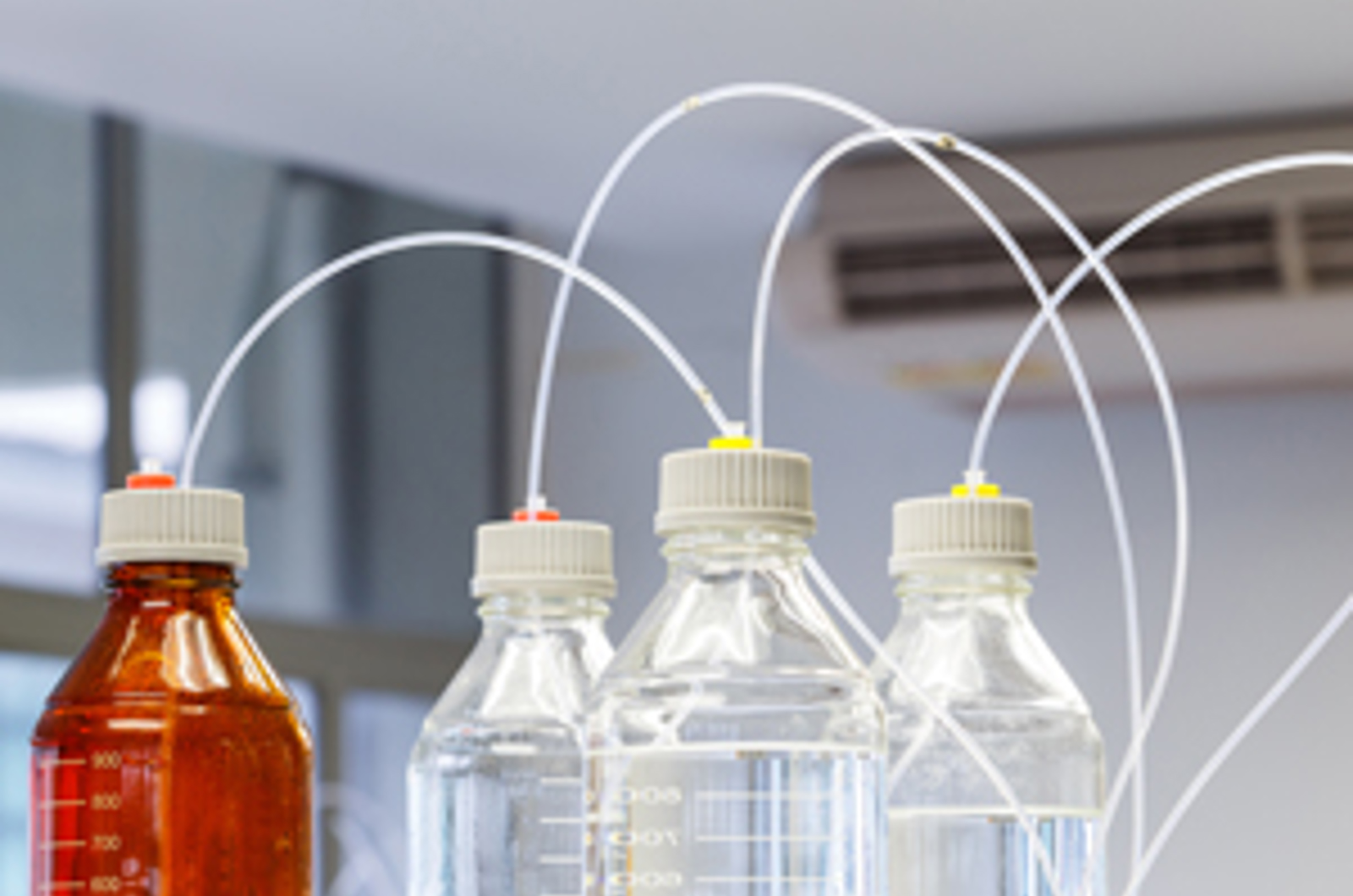
HPLC Mobile Phase Lifetimes
The question of how long you can store HPLC mobile phases is asked on a regular basis to the CHROMacademy technical team. Here are our general guidelines.
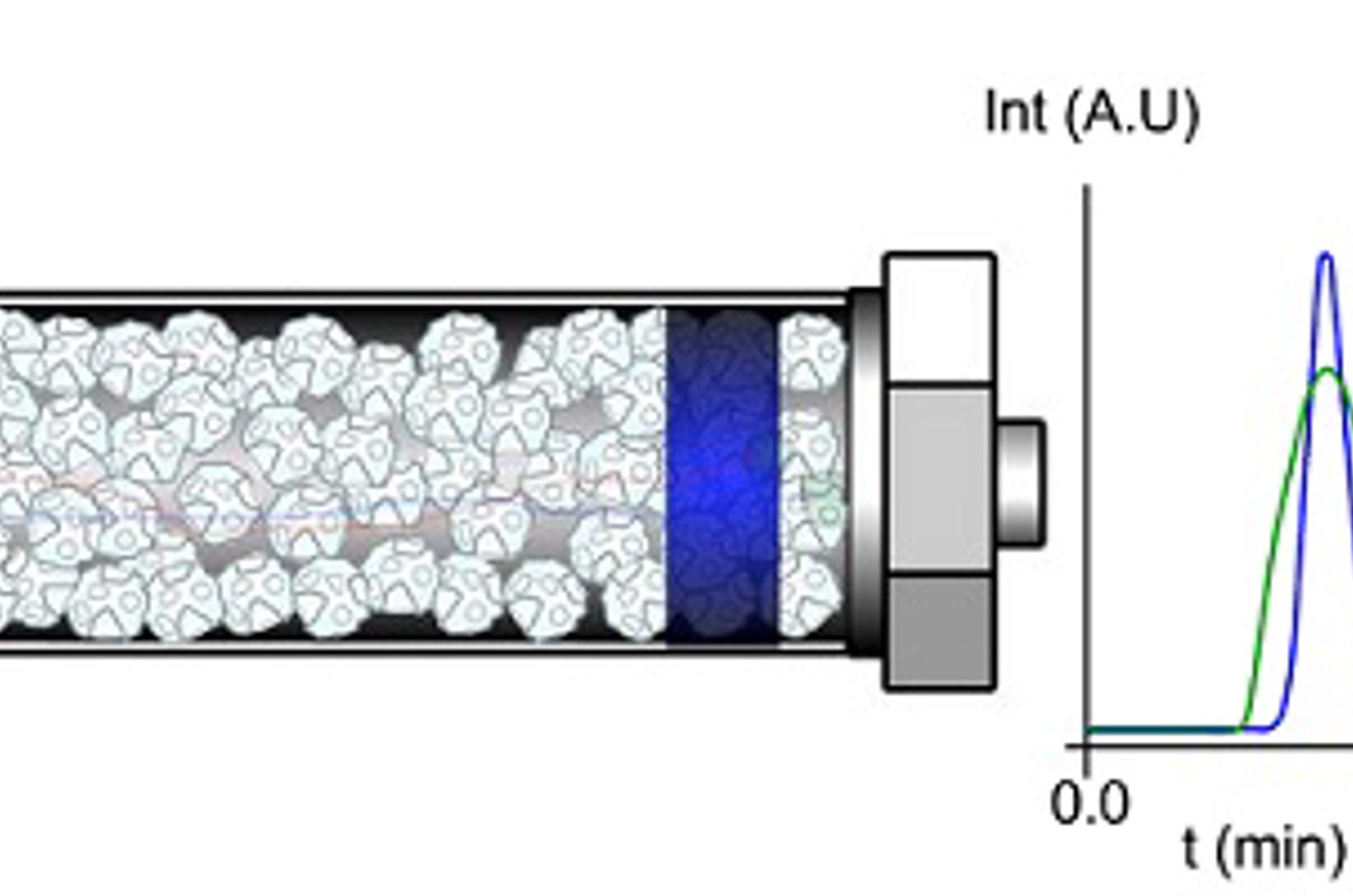
CHROMtalks - Fundamentals and Principles of Extra Column Band Broadening in UHPLC: A Tutorial
After introducing the importance and consequences of extra column dispersion, this webcast will highlight the different contributions to extra column broadening occurring in modern UHPLC instrumentation. Methodologies and the pros and cons to estimate the amplitude of extra column dispersion will be critically reviewed and some easy hands-on tests to reduce and optimize your system configuration will be provided.
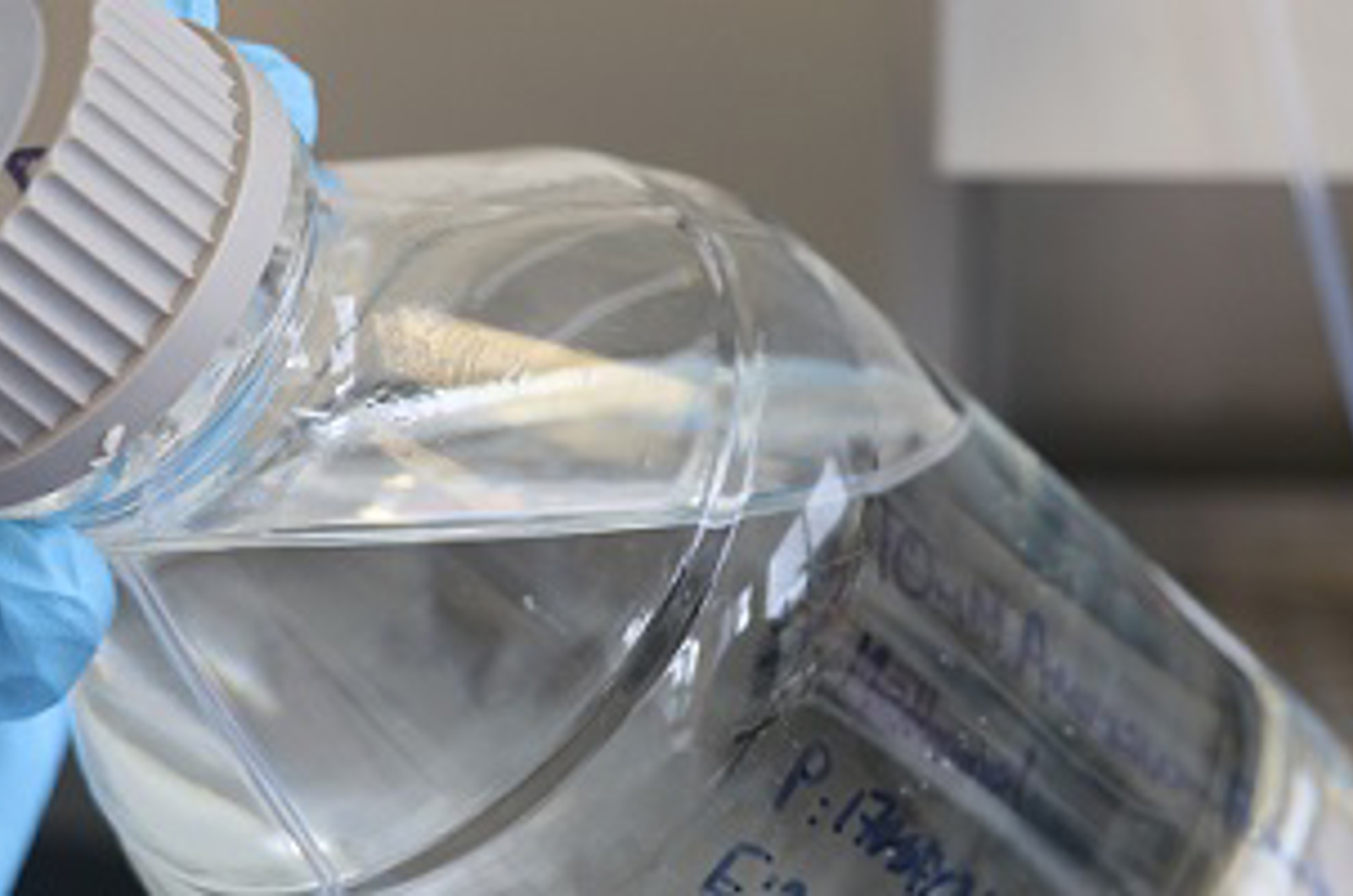
20 Steps to a Reliable Mobile Phase - Q & A
With the mobile phase being integral to the HPLC method it needs to be reliable every time. Which is why there are always lots of questions around mobile phase preparation and design to make sure we get it right first and every time.